Research Article
Volume 1 Issue 1 - 2018
Dietary Supplement Based on Pinus Radiata Seed Oil Modifies the Inflammatory Profile of Visceral Adipose Tissue in A Murine Model of Diet-Induced Obesity
1Departamento de Bioquímica Clínica e Inmunología, Facultad de Farmacia, Universidad de Concepción
2Laboratorio de Química de Productos Naturales, Departamento de Botánica, Facultad de Ciencias Naturales y Oceanográficas, Universidad de Concepción
3Unidad de Desarrollo Tecnológico, Universidad de Concepción
4Departamento de Ciencias Animales, Facultad de Ciencias Veterinarias, Universidad de Concepción
2Laboratorio de Química de Productos Naturales, Departamento de Botánica, Facultad de Ciencias Naturales y Oceanográficas, Universidad de Concepción
3Unidad de Desarrollo Tecnológico, Universidad de Concepción
4Departamento de Ciencias Animales, Facultad de Ciencias Veterinarias, Universidad de Concepción
*Corresponding Author: Daniel Duran-Sandoval, Departamento de Bioquímica Clínica e Inmunología, Facultad de Farmacia, Universidad de Concepción. Barrio Universitario s/n Concepción, Chile.
Received: October 31, 2018; Published: December 04, 2018
Abstract
Obesity is a major public health problem, both globally and particularly in our country. Therefore, all the studies that allow to understand the biological mechanisms related to the aetiology and development of obesity are of clinical and economic interest.
In this study, we analysed some of the properties of a product based on Pinus radiata seed oil (SupD) and its effect on visceral adipose tissue patches in relation to the decrease in weight gain observed in a murine model of obesity.
Our results suggest that the consumption of SupD promotes the hyperplastic growth of small adipocytes, increased vascularization in the visceral adipose tissue and favours a local anti-inflammatory environment. These observations are in agreement with the systemic metabolic effects observed in vivo, suggesting that the consumption of this product based on Pinus radiata seed oil could be clinically relevant in the control of human obesity.
Keywords: Obesity; Inflamation; Macrophages; Acid pinolenic
Abbreviations: SupD: Product based on Pinus radiata seed oil; PNA: Acid Pinolenic; PNO: Pinus nut oil
Introduction
Obesity is a pathology of high prevalence worldwide and has increased significantly since the '80s [1] [2]. Chile has one of the highest rates of childhood and juvenile obesity in the region [3]. In addition, the results of the Chilean National Health Survey 2010 [4] and 2016-2017 [5], indicated that 66.7% of the Chilean population over 15 years old presents obesity and morbid obesity, with a significant increase in the entire population, mostly in women, in recent years. The Burden of Disease Study carried out by the Chilean Ministry of Health in 2007 indicates that overweight and obesity are responsible for 7,877 deaths (9.1% of the total) in our country [6], evidencing the importance of considering the social determinants of health in the construction of public policies [7] and improve the life expectancy of our population.
Obesity is a consequence of the deregulation between the intake, expenditure and storage of energy, which may be influenced by different biological, behavioural and environmental factors. In this sense, the adipose tissue (AT) is a fundamental metabolic organ in the energy homeostatic regulation of the whole body among others through the secretion of several hormones, cytokines and metabolites that control the systemic energy balance, regulating appetite signalling through the central nervous system and peripheral tissues [8]. In response to changes in nutritional status, TA undergoes dynamic remodelling, including quantitative and qualitative alterations of resident cellular components, which include cellular differentiation processes [14] with proliferative capabilities in vivo [9].
The obvious changes in obesity would be closely related to the function of the AT, which include changes in the size and number of adipocytes in the tissue. This would affect the microenvironment of the fatty tissue, which may trigger a chronic inflammatory process and metabolic disorders that would affect the processes of lipogenesis and lipolysis [9]. Thus, obesity has been related to an increased risk of heart failure [10], arrhythmias [11] and development of insulin resistance [12], all in association with the high cardiovascular diseases risk in these individuals [13].
It is accepted that the immune cells residing in the tissues, particularly those resident in the AT, play a fundamental role in the regulation of obesity-induced inflammation. The AT contains most types of immune cells, which increase in number and level of activation, particularly macrophages, in obesity. Neutrophils, Th1Cd4+, Cd8+ cells, dendritic cells and mast cells can also be found. In addition, it is possible to found anti-inflammatory cells but is obesity that would tilt the balance towards a more inflammatory state [15]. Therefore, inflammation induced by obesity may be a different type of inflammation, that is, it is the result of overnutrition and activated stress pathways that lead to abnormal metabolic homeostasis (e.g., high lipid levels, FFA, glucose or reactive oxygen substances) [15].
It is known in the literature that nuts have a low content of saturated fats (less than 7%) and are also rich in unsaturated fats, considering them as potentially useful sources for the treatment of obesity [17]. An example of this are studies that have shown that black soybean seed extracts reduce serum glucose concentration and sensitize insulin in the model of diabetic mice [18].
The main difference between the chemical composition of the FFA present in seeds or nuts corresponds essentially to the significantly higher presence of ω3, ω4 and ω6 C18 and C20 polyunsaturated fatty acids (PUFAs). Specifically, in this type of vegetable sources, there is usually a high content of linolenic acid, pinolenic acid, coniferonic acid and sciadonic acid, among others. [19]. Among them, one of the most studied at present corresponds to Pinolenic acid (PNA) found in different species of pinaceas, being the main fatty acid present in pine oil. Seeds and pine oil have been consumed for years by several cultures in many parts of the world, because of its blood cholesterol-lowering and appetite suppressants effects. Several studies in animals have shown that dietary supplementation with pine oil, specifically from Pinus koraiensis, decreases blood lipids, reduces blood pressure and affects the metabolism of lipoproteins, but the mechanism by which it can cause these effects is not understood [16, 19, 20]. The chemical structure of PNA is very similar to its positional isomer gamma-linolenic acid (GLA), which has been shown to have beneficial effects decreasing inflammation related to some chronic diseases [21].
A study in rodents showed that consumption of a high-fat diet supplemented with pinus nut oil (PNO) was associated with lower weight gain and lower intramuscular triglyceride content compared to control animals. [20]. Studies in similar in vivo models using Pinus koraiensis oil have shown an improvement in parameters of glucose tolerance and systemic response to insulin [22, 23]. On the other hand, PNO promotes overexpression of genes such as PPARα/PPARδ and the phosphorylation of AMPK in in vitro assays, suggesting that PNA could be a potent dual ligand for PPARα/PPARδ [20]. Thus, the metabolic effects associated with the use of PNO can be mediated by the agonist effect of PNA on PPAR.
With funds from the National Commission of Scientific and Technological Research (CONICYT) we developed a project (FONDEF D11i1067) that allowed us to obtain a patented product based on Pinus radiata seed oil called SupD [62]. This product was administered in an in vivo murine model of diet-induced obesity. The results showed that SupD promote a lower weight gain associated with a significant improvement of glucose tolerance parameters and systemic response to insulin compared with control groups [62]. Thus, given the relevance of AT in the development of metabolic manifestations in obesity, the objective of this study was to establish the effect on the structure and functionality of visceral AT isolated from a murine model of diet induced obesity treated with a supplement based on Pinus radiata seed oil (SupD). Our results suggest that the consumption of SupD promotes the hyperplastic growth of small adipocytes, increases the vascularization in the visceral adipose tissue and favours a local anti-inflammatory environment. These observations are in agreement with the systemic metabolic effects observed in vivo, suggesting that the consumption of this product based on Pinus radiata seed oil could be clinically relevant in the control of human obesity.
Materials and Methods
For our study we used previously isolated tissue from an animal test conducted in the context of the FONDEF Project D11i1067 [62]. All procedures were conducted according to the guide for the care and use of laboratory animal(https://grants.nih.gov/grants/olaw/Guide-for-the-Care-and-use-of-laboratory-animals.pdf) and approved by National Commission for Scientific and Technological Research (CONICYT) Ethics Committee.
In summary, ten to twelve week-old outbred female C57BL/6J mice (n= 35) purchased from the University of Chile's bioterium were housed in a conventional animal facility maintained at 25±1°C under a 12h light/12h dark photoperiod, with free access to water and fed ad libitum with high-fat diet (HFD) D12451 (45% fat, Open Source Diets, Research Diets, Inc. New Brunswick, NJ USA) in pellet. After four months, animals achieved an obese phenotype evidenced by a significant increase in weight above 40% compared to the baseline associated with a significant increase in adipose mass. Thus, were segregated according to weight and 6h fasting glycaemia into 5 groups (7 mice each), and randomized according to the following treatments:
• Group 1: HFD diet without supplement.
• Group 2: HFD diet supplemented with 15% w/w Glycine max oil
• Group 3: HFD diet supplemented with 15% w/w Pinnothin®
• Group 4: HFD diet supplemented with 15% w/w Pinus radiata seed oil
• Group 5: HFD diet supplemented with 15% w/w SupD
• Group 1: HFD diet without supplement.
• Group 2: HFD diet supplemented with 15% w/w Glycine max oil
• Group 3: HFD diet supplemented with 15% w/w Pinnothin®
• Group 4: HFD diet supplemented with 15% w/w Pinus radiata seed oil
• Group 5: HFD diet supplemented with 15% w/w SupD
Each group of animals was fed ad libitum with their respective diet for the following 3 months.
Tissue samples
After the treatment period, the animals were fasted for 12h at the beginning of the dark cycle to establish physiological baseline and then sacrificed through exsanguination under sevoflurane anesthesia (Sojourn®, Piramal Critical Care Inc. USA). Euthanasia was performed by cervical dislocation. Subcutaneous and visceral adipose tissues were isolated from each animal, weighed and frozen in liquid nitrogen and then stored at -80°C for further analysis. Additionally, samples from visceral and subcutaneous adipose tissue were fixed in 4% w/v paraformaldehyde in PBS (Saline phosphate buffer) 1x (Merck) for 48 hours. Followed, the samples were processed in a Citadel 1000 (Shandon) automatic processor for 13 hours, under standardized protocols from the Histopathology Laboratory (Faculty of Veterinary Sciences, University of Concepción), and embedded in paraffin in a modular inclusion center (Microm AP280). Formaldehyde fixed-paraffin embedded (FFPE) tissue samples were cut in a Multicut 2045 (Leica) microtome at 4 μm thickness. Cuts were stained with Hematoxylin & Eosin (H&E), according to AFIP (Armed Forces Institute of Pathology) protocols, for morphological evaluation, infiltration distribution and quantification of the area for 100 adipocyte cells (microscope magnification 100x). The images were analyzed in a photomicroscope under an Axioscop 40 Carl Zeiss image processor, using Axiovision Release 4.6 software.
After the treatment period, the animals were fasted for 12h at the beginning of the dark cycle to establish physiological baseline and then sacrificed through exsanguination under sevoflurane anesthesia (Sojourn®, Piramal Critical Care Inc. USA). Euthanasia was performed by cervical dislocation. Subcutaneous and visceral adipose tissues were isolated from each animal, weighed and frozen in liquid nitrogen and then stored at -80°C for further analysis. Additionally, samples from visceral and subcutaneous adipose tissue were fixed in 4% w/v paraformaldehyde in PBS (Saline phosphate buffer) 1x (Merck) for 48 hours. Followed, the samples were processed in a Citadel 1000 (Shandon) automatic processor for 13 hours, under standardized protocols from the Histopathology Laboratory (Faculty of Veterinary Sciences, University of Concepción), and embedded in paraffin in a modular inclusion center (Microm AP280). Formaldehyde fixed-paraffin embedded (FFPE) tissue samples were cut in a Multicut 2045 (Leica) microtome at 4 μm thickness. Cuts were stained with Hematoxylin & Eosin (H&E), according to AFIP (Armed Forces Institute of Pathology) protocols, for morphological evaluation, infiltration distribution and quantification of the area for 100 adipocyte cells (microscope magnification 100x). The images were analyzed in a photomicroscope under an Axioscop 40 Carl Zeiss image processor, using Axiovision Release 4.6 software.
Antibodies
The following antibodies were used to detect cytokines by means of immunohistochemistry: Anti IL-6 (polyclonal rabbit; Thermofisher), anti-IL-10 (Polyclonal goat, R&D System), anti TNF-α (monoclonal mouse, Invitrogen), anti IFN -g (polyclonal goat; R&D System). The anti-Factor von Willebrand antibody (polyclonal rabbit, Dako) was used for endothelial epithelial identification. Anti CD68 (monoclonal mouse, Santa Cruz) and anti CD3 (polyclonal rabbit, Dako) were used as surface markers. For the phenotypic identification of macrophages, anti iNOS (for M1, monoclonal mouse, Thermofisher) and anti Arginase 1 (for M2, polyclonal rabbit, Santa Cruz) antibodies were used. IgG isotypes were used as negative controls.
The following antibodies were used to detect cytokines by means of immunohistochemistry: Anti IL-6 (polyclonal rabbit; Thermofisher), anti-IL-10 (Polyclonal goat, R&D System), anti TNF-α (monoclonal mouse, Invitrogen), anti IFN -g (polyclonal goat; R&D System). The anti-Factor von Willebrand antibody (polyclonal rabbit, Dako) was used for endothelial epithelial identification. Anti CD68 (monoclonal mouse, Santa Cruz) and anti CD3 (polyclonal rabbit, Dako) were used as surface markers. For the phenotypic identification of macrophages, anti iNOS (for M1, monoclonal mouse, Thermofisher) and anti Arginase 1 (for M2, polyclonal rabbit, Santa Cruz) antibodies were used. IgG isotypes were used as negative controls.
Immuno histochemical assays
The FFPE samples were cut at 4 μm, dewaxed and hydrated. Then, the endogenous peroxidase was blocked by incubation in hydrogen peroxide 0.3% v/v in methanol at room temperature for 30 minutes. For antigenic unmasking, necessary for all cytoplasmic label antibodies, sample cuts were incubated in EDTA pH 8.0 (Diagnostic biosystem) at 96 ° C in the microwave for 20 minutes. Then, slices were washed in 1x PBS, and incubated with normal horse serum at 3% v/v for protein blocking. Then, the samples were separately incubated overnight with each antibody (1:100 in Diamod Diluent Antibody, Cell marque) at 4°C. Immunostains were performed using labeled polymer (Immpres universal reactive, Vector) for one hour at room temperature, and then washed three times in 1x PBS for ten minutes. Followed, 3,3 'diaminobenzidine tetrathydrochloride (DAB; Immpact DAB, Vector) was used as a substrate. Finally, samples were counterstained with hematoxylin, dehydrated and mounted with permanent assembly medium Entellán (Merck) for further observation in a photomicroscope under an Axioscop 40 Carl Zeiss image processor.
The FFPE samples were cut at 4 μm, dewaxed and hydrated. Then, the endogenous peroxidase was blocked by incubation in hydrogen peroxide 0.3% v/v in methanol at room temperature for 30 minutes. For antigenic unmasking, necessary for all cytoplasmic label antibodies, sample cuts were incubated in EDTA pH 8.0 (Diagnostic biosystem) at 96 ° C in the microwave for 20 minutes. Then, slices were washed in 1x PBS, and incubated with normal horse serum at 3% v/v for protein blocking. Then, the samples were separately incubated overnight with each antibody (1:100 in Diamod Diluent Antibody, Cell marque) at 4°C. Immunostains were performed using labeled polymer (Immpres universal reactive, Vector) for one hour at room temperature, and then washed three times in 1x PBS for ten minutes. Followed, 3,3 'diaminobenzidine tetrathydrochloride (DAB; Immpact DAB, Vector) was used as a substrate. Finally, samples were counterstained with hematoxylin, dehydrated and mounted with permanent assembly medium Entellán (Merck) for further observation in a photomicroscope under an Axioscop 40 Carl Zeiss image processor.
Statistic analysis
The statistical analysis was performed using Graph Pad Prism® v5.03 software (Graph Pad Software, Inc., California, USA). The Gaussian distribution of the results was evaluated by the D'Agostino & Pearson test. For the variance analysis, a one-tailed ANOVA test was performed followed by a Benferroni multiple comparison test. For non-Gaussian distributions, nonparametric analyzes were used: Kruskal-Wallis test followed by Dunn's multiple comparison test. Values p < 0.05 were considered significant.
The statistical analysis was performed using Graph Pad Prism® v5.03 software (Graph Pad Software, Inc., California, USA). The Gaussian distribution of the results was evaluated by the D'Agostino & Pearson test. For the variance analysis, a one-tailed ANOVA test was performed followed by a Benferroni multiple comparison test. For non-Gaussian distributions, nonparametric analyzes were used: Kruskal-Wallis test followed by Dunn's multiple comparison test. Values p < 0.05 were considered significant.
Results
The consumption of a dietary supplement based on Pinus radiata seed oil (SupD) decreases the mass of adipocyte patches in a murine model of diet-induced obesity. Having previously established that supplementation with Pinus radiata seed oil causes a positive metabolic effect in a murine model of diet-induced obesity, evidenced by decreased weight gain, increased glucose tolerance and systemic sensitivity to insulin compared to a non-supplemented control groups, it was proposed to study whether the effect of Pinus radiata seed oil is on the structure and functionality of white adipose tissue.
As a first observation, it was shown after necropsy that the lowest weight gain in animals supplemented with Pinus radiata oil was associated with a decrease in the mass of adipocyte patches (FIG1). In fact, compared to the control supplemented with soybean oil (C-), a lower mass of subcutaneous and visceral TA is evidenced in the group treated with SupD, a decrease that is significant in the case of visceral adipose tissue (FIG1B). It should be noted that supplementation with Pinothin® (C +) is associated only with a discrete non-significant decrease in visceral patches without major effect in subcutaneous patches, considering the effects described in decreased weight gain. Although the group supplemented with 15% Pinus radiata oil shows a tendency to decrease the mass of both subcutaneous and visceral adipose patches, this was not significant. Therefore, samples from this group will not be used in subsequent analyses.
The consumption of SupD decreases the size of adipocytes in visceral adipose tissue
Considering that visceral TA patches showed significant changes with the dietary supplementation with Pinus radiata seed oil, it was established to measure and to compare cell size and distribution in these tissues by histological analysis. In a first stage, 4 mm cuts were obtained from 10% w/v formalin-fixed paraffin embedded (FFPE) tissues and stained with Hematoxylin & Eosin (H&E) stain for analysis in an optical microscope.
Considering that visceral TA patches showed significant changes with the dietary supplementation with Pinus radiata seed oil, it was established to measure and to compare cell size and distribution in these tissues by histological analysis. In a first stage, 4 mm cuts were obtained from 10% w/v formalin-fixed paraffin embedded (FFPE) tissues and stained with Hematoxylin & Eosin (H&E) stain for analysis in an optical microscope.
As shown in Figure 2A, the non-supplemented obese basal control group (Basal) shows a wide range of adipocyte sizes, with a higher percentage of frequency between 3,000 and 10,000 μm2/cell, the latter considered hypertrophic, which also involved a smaller number of cells per field (FIG3A). This cellular distribution is accompanied by mononuclear cells, which are distributed mainly surrounding crown-like structures (CLS) (FIG 4A, B) and blood vessels of smaller diameter, which will need to be evidenced by specific markers.
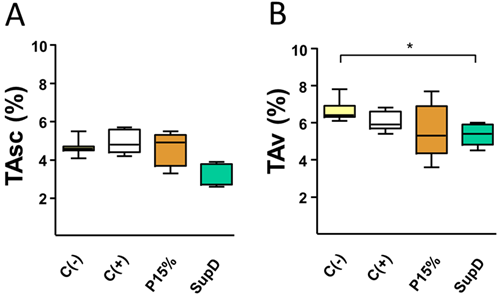
Figure 1: Oil supplement modifies adipose tissue mass in vivo. Percentages of relative weight of A) subcutaneous AT (TAsc) and B) visceral AT (TAv) of the different groups under study. Supplement with 15% soybean oil (C-), 15% Pinothin® (C +), 15% Pinus radiata oil (P15%) and 15% SupD.
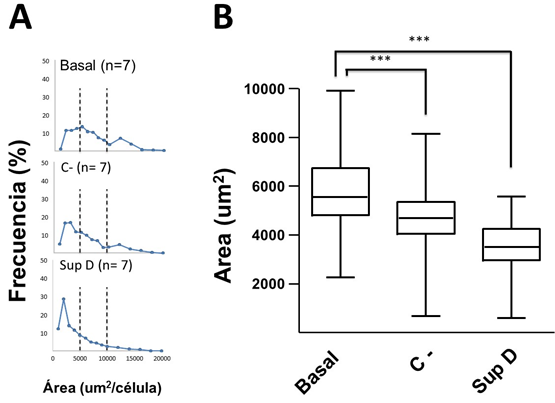
Figure 2: Analysis of adipocyte sizes. A) Frequency of adipocytes according to size. Dotted line establishes adipocyte limit values of small ( < 5000 µm2/cell) and large size (> 10000 µm2/cell). B) Box graph of the frequencies of adipocyte sizes according to treatment. Basal = group without supplement; C- = HFD supplemented with 15% soybean oil; SupD = HFD supplemented with 15% SupD. (***) p < 0.001.
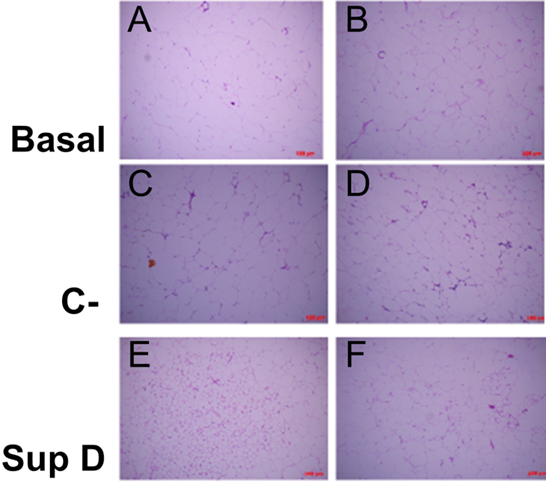
Figure 3: Scattering of cell sizes according to supplement. Increase 100x (A, C, E), increase 200x (B, D, F). Basal: Obese control without supplement; C-: HFD plus supplement 15% soybean oil; SupD: HFD plus supplement 15% SupD.
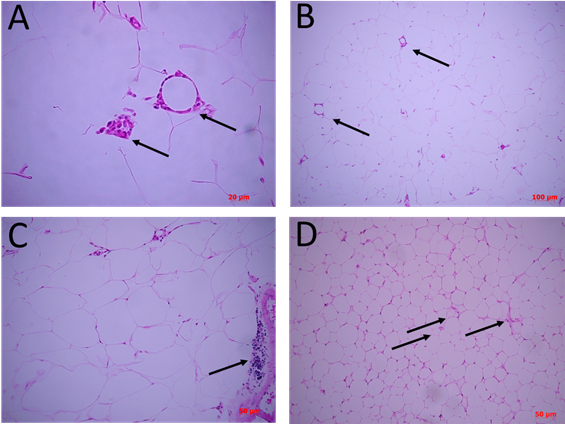
Figure 4: Differential cellular structures between supplements. Cuts of 4 µm from FFPE tissues, stained with H&E stain and observed under optical microscope. In A) there is adipose tissue basal control without supplement. Arrows indicate crown structures (CLS) at 200X and a panoramic view using a smaller objective of the same cuts (B). C) adipose tissue under supplement with 15% soybean oil. Arrow indicate focal mononuclear infiltrative zones. D) adipose tissue under supplementation with 15% SupD. Arrows indicate areas with micro vacuolated adipocytes and possible structures of small vessels.
Gen | Primers | Amplicon size (bp) | MT (ºC) | Access number |
PPAR-g | F- CACTGCCTATGAGCACTTCAC | 55 | 55 | NM_011146.3 |
R- AATGGCATCTCTGTGTCAACC | 55 | |||
b-2m | F- TGCAGAGTTAAGCATGCCAGTA | 62 | 57 | NM_009735.3 |
R- CATGTCTCGATCCCAGTAGACG | 56 | |||
UCP1 | F- AAGTCCGCCTTCAGATCCAA | 51 | 55 | NM_009463.3 |
R- ACCTAATGGTACTGGAAGCCT | 54 | |||
Hprt | F- TGGACAGGACTGAAAGACTTGCT | 52 | 57 | NM_013556.2 |
R- TGGCCTCCCATCTCCTTCAT | 56 |
Table 1: Description of genes used for q-PCR analysis.
The adipocytes of the group supplemented with soybean oil (C-) were those that showed a high dispersion of ranges of sizes, between 1,000 to 8,000 μm2/cell (FIG2A), which were significantly lower compared to Basal group (FIG2B). Despite this, the number of cells per field was variable (FIG3 C, D). In addition, an important mononuclear cell infiltrate was observed, more focused on certain sectors of the visceral adipose tissue (FIG4C).
The obese group supplemented with 15% SupD, clearly showed a high frequency of adipocytes of smaller size (FIG2A), being found at an average around 4,000 μm2/cell, so it was possible to find a greater number of cells per field (FIG3 E, F). The size of the adipocytes in this group was significantly lower than that observed in the non-supplemented obese basal group (FIG2B). Unlike the non-supplemented obese basal control, the sizes of the SupD group were found among the lowest values of the experimental model (800 to 6,000 μm2/cell). However, it was interesting to note that these small adipocytes are grouped in niches of small adipocytes (FIG3 E, F), which are accompanied by some mononuclear infiltrative cells and structures that resemble blood vessels. On the other hand, the CLS in these tissues are practically non-existent (FIG4 D).
SupD decreases the number of mononuclear cells that infiltrate visceral adipose tissue.
To elucidate the cell type corresponding to the mononuclear infiltrate observed in the AT studied, we used specific antibodies against surface markers: anti-CD68, for the identification of macrophages; anti-CD3, for identification of T lymphocyte cells.
To elucidate the cell type corresponding to the mononuclear infiltrate observed in the AT studied, we used specific antibodies against surface markers: anti-CD68, for the identification of macrophages; anti-CD3, for identification of T lymphocyte cells.
In the Basal group, without supplementation, we observed that there is a high and almost exclusive presence of CD68 immunoreactive cells resident near to larger adipocytes, but preferably as part of CLS structures surrounding sometimes necrotic adipocytes (FIG 5 A, B). The negative control (C-), supplemented with 15% soybean oil, also showed areas of cell infiltration where some responded to the CD68 marker, most of them close to CLS (FIG 5 D) but qualitatively in smaller quantity than in the Basal group (FIG 5 C). However, the cells of the focal infiltrate, that characterized this group, responded mainly to the CD3 marker, suggesting that the mononuclear infiltrate corresponded to T lymphocytes (FIG5 J, K).
Regarding our experimental group, dietary supplementation with SupD was associated with a visceral TA with less cellular infiltration, an infiltrate that was immunoreactive to CD36 concordant with macrophages (FIG5 E, F). As there are fewer CLS structures in this group, most of the macrophages are resident and accompanying the smaller adipocyte niches previously described (FIG 5 E, F). Table 2 shows the average count of CD36+ macrophage like cells between goups. We can observe that the SupD group shows the least amount of infiltrative cells in the stroma, reactive to CD36, and corresponding to macrophages.
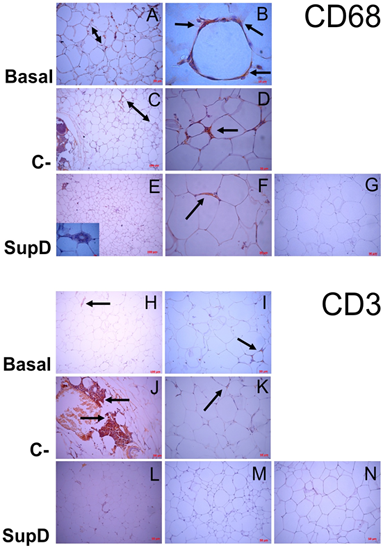
Figure 5: Mononuclear infiltrate evaluation. Immunostaining was performed against CD68 (Macrophages; A-G) and CD3 (T-lymphocytes; H-N). Basal: Obese control without supplement; C-: supplement 15% soybean oil; SupD: Supplement 15% SupD. A) Panoramic increase 200x, presence of infiltrate (Double arrow). B) Hypertrophic adipocyte surrounded by macrophages (arrow) C) Panoramic 100x, lower presence of macrophage infiltrate (double arrow) D) 400x magnification, small foci of macrophages. (Arrow) E) Inside box, 1000x magnification, show necrotic adipocyte surrounded by macrophages. F) Arrow indicates macrophages around larger adipocytes. H) to K) Lymphocyte infiltrate (Arrow); L), M) practically negative to lymphocyte marker. G) and N) Negative controls, respectively.
Obese Control without supplementation Average ± SD (values per sample) | Negative control 15% soybean oil Average ± SD (values per sample) | SupD 15% supplement D Average ± SD (values per sample) |
328 ± 106 (215, 425, 345) | 142 ± 19 (150, 156, 120) | 128 ± 57 (79, 114, 190) |
Table 2: Average count of macrophage cells in visceral adipose tissue. After immunostaining against CD36, the positive cells were counted in 10 random fields with 10x objective, in three different samples of each group.
SupD increases the relative vascularity in visceral adipose tissue
As previously mentioned, the stromal structure of the adipose tissue suggested modifications in the distribution of blood vessels depending on the experimental group studied. Therefore, using an anti-VIII von Willembrand factor, marker of vascular endothelium, it could be observed that the group fed with SupD show a greater relative vascularity compared with the obese Basal group, without treatment, and that negative control (C-), supplemented with 15% soybean oil (FIG6). In addition, it was evidenced that blood vessels in the SupD group are near to the small adipocyte niches that have been characteristic in this group. The obese Basal control group showed the lowest presence of blood vessels in the stroma (FIG6 A).
As previously mentioned, the stromal structure of the adipose tissue suggested modifications in the distribution of blood vessels depending on the experimental group studied. Therefore, using an anti-VIII von Willembrand factor, marker of vascular endothelium, it could be observed that the group fed with SupD show a greater relative vascularity compared with the obese Basal group, without treatment, and that negative control (C-), supplemented with 15% soybean oil (FIG6). In addition, it was evidenced that blood vessels in the SupD group are near to the small adipocyte niches that have been characteristic in this group. The obese Basal control group showed the lowest presence of blood vessels in the stroma (FIG6 A).
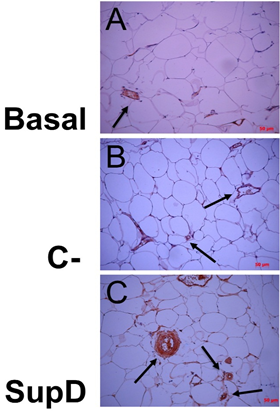
Figure 6: Associated vascularity, according to supplement. Immuno histochemical images against FVIII von Willenbrand Factor. Basal: Obese control without supplement; C-: Supplement 15% soybean oil; Sup D: Supplement 15% SupD.
SupD helps to establish an anti-inflammatory environment in visceral adipose tissue.
Given that the monocyte infiltrate in AT was mainly identified as macrophages, it was necessary to verify if these populations belong to a pro-inflammatory or anti-inflammatory phenotype. This aspect is fundamental to determine a relationship between the type of food supplement and the possible function of this macrophage infiltrate in the different experimental conditions. For this reason, the same samples previously analysed were studied by immunohistochemistry, including markers involved in inflammation, in particular: IL-6, TNF-α and IFN-γ.
Given that the monocyte infiltrate in AT was mainly identified as macrophages, it was necessary to verify if these populations belong to a pro-inflammatory or anti-inflammatory phenotype. This aspect is fundamental to determine a relationship between the type of food supplement and the possible function of this macrophage infiltrate in the different experimental conditions. For this reason, the same samples previously analysed were studied by immunohistochemistry, including markers involved in inflammation, in particular: IL-6, TNF-α and IFN-γ.
IL-6 was the cytokine that had an intense immunoreaction in the obese Basal group (FIG 7 A, B). However, a high relative intensity is evidenced in the SupD group compared to the other groups (FIG 7 D, E). It is also observed that immunostaining diffuses into the adipose tissue stroma, probably associated with the fact that macrophages are not the only cells in this tissue that release IL-6.
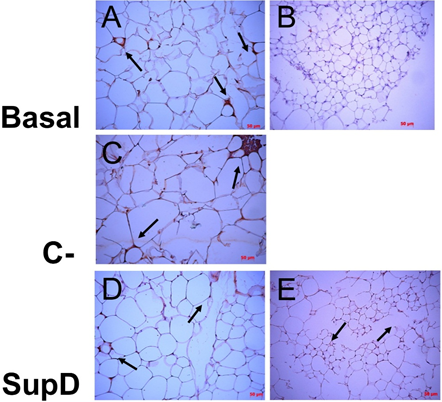
Figure 7: Immunoreactivity to IL-6. Immunohistochemistry images against IL-6. The arrows mark areas of immunoreactivity. Basal: Obese control without supplement; C-: Supplement 15% soybean oil; Sup D: Supplement 15% SupD. B) negative control E) panoramic view.
In the case of TNF-α and IFN-γ it was observed that the obese basal group presents an intense immunoreaction to these cytokines, especially in the CLS structures, more abundant in this group (FIG8, FIG9). The negative control (C-), supplemented with 15% soybean oil, shows an intermediate immunoreactivity profile, with IFN-γ being the one with the highest intensity in the infiltrate areas, which, as we saw earlier, corresponded to T lymphocytes, probably responsible for its secretion (FIG9). On the other hand, it can be observed that the experimental group SupD presents a lower immunoreactivity for cytokines TNF-α and IFN-γ compared with the other groups (FIG8, FIG9).
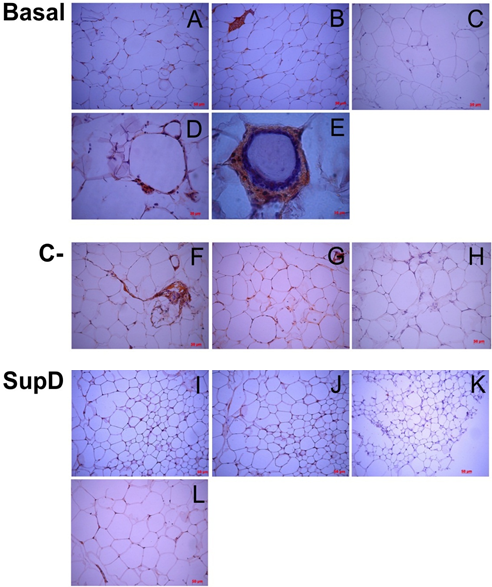
Figure 8: Immunoreactivity to TNF-α. Immunostaining was performed against TNF-α. C), H), K) Negative immunoreactive controls. Basal: Obese control without supplement; C-: Supplement 15% soybean oil; Sup D: Supplement 15% SupD.
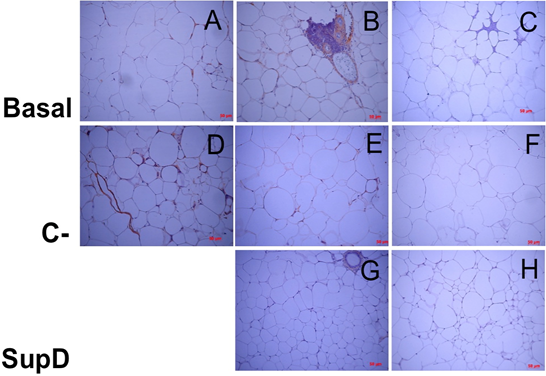
Figure 9: Inmunoreactivity against INF-. Imaging of immunohistochemistry against INF-γ. C), F and H) Negative immunoreactive controls, respectively. Basal: Obese control without supplement; C-: Supplement 15% soybean oil; Sup D: Supplement 15% SupD.
Our results suggest that the environment surrounding large adipocytes is rather pro-inflammatory. In contrast, the environment that accompanies adipocytes in the visceral AT of the group supplemented with SupD shows a lower immunoreaction to the cytokines analysed. That is why it is essential to determine the presence of anti-inflammatory cytokines to guide us if the inflammatory environment is associated with an anti-inflammatory profile induced by SupD. Thus, the same samples were subjected to the immune his to chemical protocol to visualize the presence of cells immune reactive to IL-10. It was observed that the group that had greater immunoreactivity to IL-10 was the SupD group and that, in addition, these immune reactive cells could also be found infiltrating sites near the small adipocyte niches (FIG10). In contrast, our obese baseline control was practically negative to the antibody labelling against IL-10 and the control supplemented with 15% soybean oil had a low positivity (FIG10).
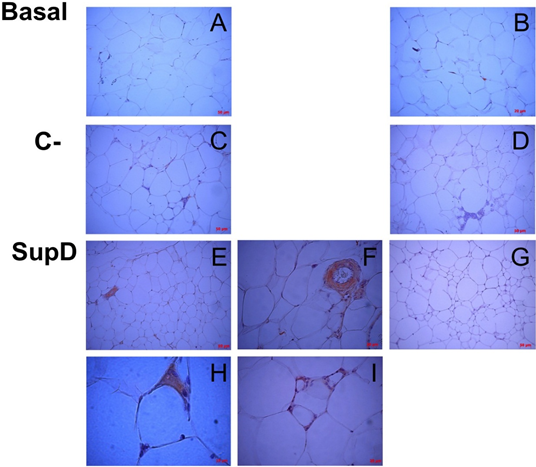
Figure 10: Inmunoreactivity against IL-10. Immunostaining was performed against IL10. Each panel details particular areas of the fabric cut. B), D), G) Negative immunoreactive controls, respectively. Basal: Obese control without supplement; C-: Supplement 15% soybean oil; Sup D: Supplement 15% SupD.
SupD contributes to the polarization of macrophages towards M2.
Given the differences in the profile of cytokines observed in the AT, depending on the group studied, probably the population of macrophages that infiltrate the AT also have particular characteristics. In order to differentiate these populations of macrophages, the identification was made with monoclonal antibody against iNOS (inducible nitric oxide synthase), to identify the population of macrophages M1, corresponding to a pro-inflammatory phenotype, and anti-Arginase 1 antibody as a cytoplasmic marker of the macrophage population with M2 phenotype, anti-inflammatory.
Given the differences in the profile of cytokines observed in the AT, depending on the group studied, probably the population of macrophages that infiltrate the AT also have particular characteristics. In order to differentiate these populations of macrophages, the identification was made with monoclonal antibody against iNOS (inducible nitric oxide synthase), to identify the population of macrophages M1, corresponding to a pro-inflammatory phenotype, and anti-Arginase 1 antibody as a cytoplasmic marker of the macrophage population with M2 phenotype, anti-inflammatory.
In this context, it was observed that the group with the highest population of macrophages polarized towards M1 corresponds to the obese basal group without supplementation (FIG11A) and that they are also the same that surround the CLS (FIG11 B, C). The negative control supplemented with 15% soybean oil has an intermediate profile since not all the cells that infiltrate the tissue correspond to macrophages and also not all the macrophages present are M1, and those that are positive for iNOS are found to be part of these CLS (FIG11 E). In contrast, the group supplemented with 15% SupD presents a population of macrophages mostly polarized towards M2 in an in situ observation (FIG12), which is evident in comparison to the other experimental groups analysed.
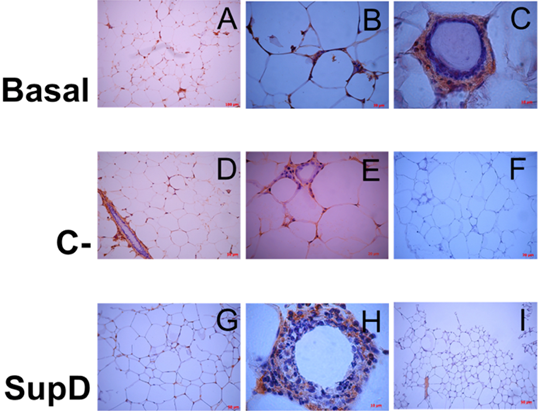
Figure 11: Inmunoreactivity against iNOS. Immunostaining was performed against iNOS. Each panel shows particular areas from tissue cuts. Basal: Obese control without supplement; C-: Supplement 15% soybean oil; Sup D: Supplement 15% SupD.
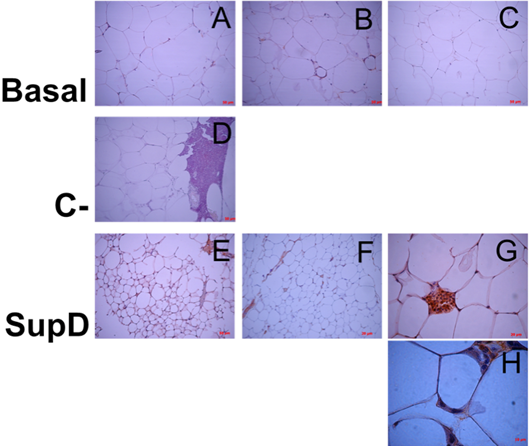
Figure 12: Inmunoreactivity against Arginase 1. Immunostaining was performed against Arginase 1. Each panel shows particular areas from tissue cuts. Basal: Obese control without supplement; C-: Supplement 15% soybean oil; Sup D: Supplement 15% SupD.
Table 3 shows an approximate average count of M1 and M2 macrophage type cells, according to experimental group. We can observe that the SupD group has a lower M1/M2 ratio than that observed in the obese Basal group and negative control (C-) treated with soybean oil. This reinforces the idea that SupD promotes an anti-inflammatory environment associated with macrophages with an M2 profile.
Group | M1 (n) | M2 (n) | M1/M2 (razón) |
Obese control without supplementation | 149 | 18 | 8,2 |
Supplement with 15% soybean oil | 92 | 32 | 2,9 |
Supplement with 15% SupD | 56 | 84 | 0,7 |
Table 3: Average count of M1 and M2 cells in visceral adipose tissue. After immunostaining against iNOS or Arginase 1, the positive cells were counted in 10 randomized fields with a 100x objective, in three samples, of three mice from each group.
Discussion
Obesity is considered an epidemic worldwide, where the search for therapeutic alternatives for its management becomes necessary. In Asia, diverse cultures consume conifer seeds or their oil as a "healthy food". In this sense, it has been shown that oil consumption obtained from certain Asian pinnaceae would have anorexigenic effects [22], suggesting this product as an alternative for the treatment of obesity. As Chile is a country with high forest industrial activity, particularly in pinacea cultivation, in 2012, with funds from the National Commission of Scientific and Technological Research (CONICYT), we generated a product based on conifer seed oil as an appetite modulator [62]. In the context of these project, we evaluated in vivo the effect of a dietary supplement based on Pinus radiata seed oil (SupD) in a murine model of diet induced obesity. Results from intervention showed that SupD produces a modification in the pattern of daily food consumption, lower weight gain at the expense of lower visceral fat mass, greater glucose tolerance and increased systemic sensitivity to insulin [62]. However, the mechanisms that explain this phenomenon are unknown until now. Given the relevance of adipose tissue in the development of metabolic manifestations in obesity, the objective of this study was to establish the effect of this supplement based on Pinus radiata seed oil (SupD) on the structure and functionality of white AT previously isolated from a murine model of obesity induced by diet supplemented with SupD. Our results suggest that the dietary consumption of SupD promotes the hyperplastic growth of small adipocytes, increases the vascularization in the visceral AT and improves a local anti-inflammatory environment. These observations are in agreement with the systemic metabolic effects observed in vivo, suggesting that the consumption of this product based on Pinus radiata seed oil could be clinically relevant in the control of human obesity.
The factors that determine the fat mass in an individual are not yet completely clear, however, it is evident that the adipose tissue mass can expand at the expense of an increase in the volume and/or the number of adipocytes. Therefore, an analysis can be made from the point of view of the relationship between the volume of fat cells and the body mass index. In this sense, there are reports that have found a positive correlation between the amount of fat mass and the volume of fat cells both in the subcutaneous patches, which represents approximately 80% of all body fat, and in the visceral fat patches that they have a strong link with the metabolic complications of obesity [24].
Supported by this idea, our results indicate that dietary supplementation with oil derived from Pinus radiata seeds (SupD) in a murine model of diet-induced obesity decreases the mass of visceral adipocyte patches due to a decrease in the size of the adipocytes, associated to the formation of niches of small adipocytes accompanied by infiltrative cells. The mechanisms that explain these results are not clear. However, the first scientific studies in relation to the incorporation in the diet of a pine seed oil, particularly the Pinus koraenensis seeds oil, already indicated that this product exerted diverse physiological functions that would be in favor of the prevention or decrease in several degenerative disorders such as hypercholesterolemia, thrombosis and hypertension, an effect attributed to its main component, the trienic acid called pinolenic acid (Pinolenic acid; PNA) [25].
Based on this background, it was plausible to propouse that PNA was responsible for the decrease in the size of visceral adipocyte patches. However, we now know that this effect is not only attributable to the PNA, since our results show that in the group supplemented with soybean oil, oil that does not contain PNA, also shows a tendency to decrease the mass of the adipocyte patches visceral, although this decrease also involves a wide dispersion of sizes of adipocytes but, on average, tend to be smaller than the control Basal group. However, soybean oil supplementation was not histologically associated with the formation of niches as in the case of the SupD group. These results suggests that molecules present in Pinus radiata seed oil, different from those present in soybean oil, including PNA, would ultimately be responsible for the cellular effects that are observed on visceral adipose tissue in our model.
As previously mentioned, SupD improves glucose tolerance and increases systemic sensitivity to insulin at the expense of lower visceral fat mass in a murine model of diet-induced obesity [62]. However, it is necessary to analyse whether the decrease in the mass of the adipocyte patches is responsible for an improvement in the metabolic parameters, or that the improvement of the metabolic parameters influences the decrease in the mass of the adipocyte patches.
The risk of metabolic complications increases not only because of the amount and location of adipose tissue, but also because of the size of your fat cells. Recent studies have suggested that there would be a relationship between adipocyte hypertrophy and insulin resistance [26]. For example, it has been shown that mRNA levels and secretion of serum amyloid A (SAA) in obese and non-obese individuals, thinly obese individuals and people treated with rosiglitazone (an insulin sensitizer drug) is elevated in human adipocytes, significantly correlating with the body mass index [27]. In addition, the decrease in serum SAA, an acute phase protein involved in inflammation, was significantly correlated with the magnitude of improvement in insulin sensitivity after weight loss [27]. In cell cultures, the treatment of vascular endothelial cells and monocytes with SAA markedly increased the production of inflammatory cytokines, for example: interleukin (IL) -6, IL-8, tumor necrosis factor alpha (TNF-α) and chemoattractant protein of monocytes 1 (MCP-1).
In addition, SAA increased basal lipolysis in the adipose tissue culture by about 47%, in this cellular model. Based on these evidences, a high body mass index due to an increase in the adipose tissue mass given to cellular hypertrophy, would help to maintain an inflammatory microenvironment, stimulating the apoptosis of adipocytes and, therefore, the establishment of a dysfunctional adipose tissue, a phenomenon that could be reversed, at least partially, with an eutrophy of adipose tissue. If we analyse our results, we can show that animals supplemented with SupD have a decrease in visceral adipocyte size. This decrease in size was statistically significant with respect to the non-supplemented obese baseline control. This evidence, together with the improvement in glucose tolerance and the increase in the systemic sensitivity to insulin observed in vivo in this group, suggests that the size of adipocytes would be directly related to the alteration of clinical markers linked to obesity, a situation that contrasts with what was observed in the obese Basal control and that occurs to a lesser extent in the other groups.
In the obese AT from human or animals, several metabolic functions are subject to changes with the size of the adipocytes. These functions include the storage and mobilization of lipids and the secretion of both leptin and TNF-α [28].
However, obesity is characterized by metabolic alterations such as insulin resistance and it is not known if the changes in the fat cells, increased in obese subjects, are only due to the hormonal and nutritional environment, or if the cellular hypertrophy per se also has a role in this adaptation [29].
The plasticity of adipose tissue includes two types of expansion: hypertrophic (larger adipocytes) and hyperplastic (increase in the number of adipocytes) expansion or both, considering that the development of tissues is not homogeneous. These mechanisms are obviously regulated by environmental and genetic factors [30]. It has been proposed that a hypertrophic adipocyte derives in a functionally abnormal adipocyte. In addition, the size of the adipocyte would also be an indicator of the life cycle of the adipocyte. Thus, according to these criteria, in general large adipocytes would be more advanced in their life cycle than smaller ones. [31]
The contribution of adipose tissue to insulin resistance may involve cell dysfunction associated with altered morphology, adipocytokine secretion profile or metabolism of adipocytes. Previous studies have indicated that the increase in size of subcutaneous adipocytes predicts the development of insulin resistance and type 2 diabetes mellitus, and that their size is negatively correlated with insulin sensitivity and correlates positively with pro-inflammatory adipocytokine secretion. [32]. Other findings point out to a primary defect in the adipose cell that is related to the development of insulin resistance in obese individuals. The findings of an improved population of small adipose cells and the diminished expression of genes related to the differentiation of fat cells, strongly suggest that the variation in the characteristics of adipose cells are independent of obesity, but probably interact with obesity, where they play an important role in the development of insulin resistance [33]. Specifically, these studies suggest that, in the context of obesity, people who cannot recruit an additional population of mature fat cells for the storage of triacylglycerol will develop insulin resistance.
In an era of high prevalence of overweight and obesity, different findings highlight the need to study adipose tissue cells as a possible mediator of insulin resistance. Recent reports have shown that insulin resistance and overexpression of genes involved in adipogenesis and inflammation would be associated with the size distribution of subcutaneous adipocytes, specifically an increase in the fraction of small cells, and not with the average diameter of the large cells [34].
In this sense, our work is a contribution, considering that in the group fed with SupD there are smaller adipocytes but, in addition, they are grouped in niches. This conformation makes us think that these smaller adipocytes would fulfill different metabolic functions or that they would be in different stages in their life cycle and, therefore, they would present different surface markers, and that the incorporation of SupD in the diet would play a role key to a beneficial phenotype change for visceral adipose tissue.
First, in adipose tissue from obese models, hypertrophic adipocytes present necrotic abnormalities, which has led to the proposition that the increase of dead adipocytes in obesity would impede the normal functions of adipose tissue and induce inflammation [35]. It has also been found that the expression of leptin would be related rather to the number and not to the size of the adipocyte in rats of the JCR-LA strain [36]. Thus, it is not entirely clear if all metabolic functions vary with cell size. Research has documented severe organic alterations and signs of oxidative stress in the hypertrophic adipocytes of genetically obese mice that is reminiscent of the inflammation that occurs in atherosclerosis, that is, the result of overnutrition with activation of stress pathways that lead to abnormal metabolic homeostasis (high levels of lipids, FFA, glucose or reactive oxygen substances) [38]. The degeneration of adipocytes results in the release of cellular content in the extracellular space and that is phagocytosed after the recruitment of macrophages, which ultimately leads to the formation of CLS [38]. Same characteristics could be seen in our study group, where our basal non-supplemented control had these characteristics including CLS structure accompanying necrotic adipocytes between large adipocytes.
The literature shows that the increase in BMI and the average size of fat cells in humans and rodents are associated with a higher density of macrophages in adipose tissue, and that large fat cells appear necrotic and is surrounded by macrophages. The increases in the white adipose tissue mass reflect the hypertrophy of the adipocytes, the hyperplasia of the adipocytes or both. The correlation of the death of adipocytes with the size of them and the associations of adipocyte hypertrophy with deregulated metabolism and increased cellular stress, suggest that hypertrophy of adipocytes could promote adipocyte death associated with obesity [37] It has been shown that the presence of macrophages in the white adipose tissue of lean and obese humans and mice is selectively located close to dead adipocytes and that the death rates of adipocytes increase drastically in obesity, potentially reflecting the cytotoxic effects of hypertrophy of the adipocytes. In addition, the death of adipocytes associated with obesity seems to imply an alternative death route that exhibits morphological features of necrosis and the profile of apoptosis caused by leukocyte. [37].
These observations provide a new framework for understanding the recruitment and function of macrophages in white adipose tissue and for clarifying the underlying causes of white adipose tissue inflammation associated with obesity. The murine model of obesity induced by diet that we used for our study, showed results that coincide with the evidence provided by the literature. Indeed, our non-supplemented obese Basal control had CLS among whose constituent cells corresponded to macrophages, identified by specific markers. The presence of these CLS decreased in the group with a diet supplemented with SupD. Thus, the supplement based on Pinus radiata seeds oil would have a beneficial effect on the improvement of the inflammatory microenvironment characteristic of obesity. In this regard, more studies will be necessary to know the mechanisms involved, for which this oil would cause this change. Consistently with what was published [54], in our study we observed an accumulation of macrophage-like cells in the obese Basal control. However, this infiltration decreased in the group whose diet was supplemented with SupD. Once again we are under a beneficial effect when incorporating oil derived from pine seeds, although details of the polarization and characterization will be discussed later.
During the expansion of fat mass in obesity, the vascularization of adipose tissue is insufficient to maintain tissue normoxia. Local hypoxia leads to an altered expression of adipocitokines, recruitment of pro-inflammatory macrophages and insulin resistance [39].
Classical studies of blood flow in vivo show a constant supply of blood per adipocyte as the animal grows. However, blood flow per unit area of adipocytes decreases with obesity, which increases the possibility that the supply of oxygen and nutrients to the adipocyte is less than optimal [40]. The vascularity of obese adipose tissue may also exhibit abnormal responses to vasodilators (eg, NO) or increased permeability. Direct measurements of oxygenation document a lower oxygen tension (pO2) with a lower capillary density in obese patients. In addition, pO2 was inversely correlated with pro-inflammatory markers, suggesting that lower pO2 in situ could lead to inflammation of AT in obesity, consistent with a causal role of low vascularization for hypoxia, fibrosis and dysfunction of adipocytes [40].
In thin subjects, macrophages in adipose tissue contribute to the regulation of tissue function, as well as remodelling. Several lines of evidence suggest that macrophages residing in AT influence angiogenesis. In mouse models, the depletion of macrophages present in AT, through the use of liposomes, reduces the structures of blood vessels in this tissue. In addition, macrophages recruited at the ends of gonadal adipose tissue promote angiogenesis during tissue growth. In particular, lymphatic endothelial receptor 1 macrophages secrete tissue remodeling factors, such as matrix metalloproteinase (MMP) -7, MMP-9 and MMP-12 that stimulate VEGF/VEGFR signalling for the formation of new blood vessels [41-43]. In our study, we found that there was a greater number of macrophage-like cells in the non-supplemented obese Basal control, compared to the other groups. In addition, we observed an increase in relative vascularity in the SupD group. There would be, therefore, a relationship between the incorporation of SupD in the diet with increased angiogenesis, which could be associated with an increase in the flow of oxygen to the tissue and, with that, help to an improvement in the infiltration of macrophage-like cells that accompany an obese adipose tissue. It will be interesting to evaluate in depth this phenomenon, to know exactly what the mechanism by which SupD exercises this change is.
A very interesting feature of the inflammatory response that arises in obesity is that it seems to be activated and focused predominantly on adipose tissue, although other metabolically critical sites may also be involved during the course of the disease. The temporal and spatial properties of the inflammatory response in the context of obesity and its complications, the target cell(s) that are critical in metabolic deregulation and the underlying molecular mechanisms, remain as unanswered but critical questions [44] A series of studies in humans and mice have suggested that the increase of the diameter of the adipose cells is associated with a greater inflammation in the adipose tissue therefore with high local concentrations of IL-6 and TNF-α in the adipose tissue. The addition of IL-6, and even more with TNF-α, induces dramatic effects in the terminal differentiation program and produces cells with reduced lipid accumulation and reduced expression of several genes of importance for normal metabolism, sensitivity and action of the insulin such as: IRS-1, GLUT4, adiponectin, lipoprotein lipase, fatty acid synthase and perilipin [45]. Our in situ analyzes showed that the group supplemented with SupD had a decreased expression of IL-6 and much more evident in the case of TNF-α. Basal and negative control groups showed a marked reaction to these molecules and, in addition, were associated with CLS, indicating that the production of certain inflammatory cytokines are linked to obesity and also to necrosis of adipocyte cells. Studies in vitro have shown that TNF-α derived from macrophages plays a critical role in the induction of inflammatory changes in adipocytes and in the release of free fatty acids that, in turn, induces inflammatory changes in macrophages, at least in part through the MAP kinase pathways. In addition, it has been shown that the Estromal Vascular Fraction (EVF) in ob/ob mice, a monogenic model of obesity, exhibit a more abundant expression of TNF-α as well as of the macrophage marker F4/80 in relation to wild type mice.
These observations suggest that EVF in obese mice has the potential to induce inflammatory changes in adipocytes due to a larger population of macrophages [46]. This could be the scenario we find in the case of the non-supplemented Basal obese group, where the increase in pro-inflammatory cytokines such as IL-6 and TNF-α is more evident. Therefore, SupD would be exerting an effect on the expression profile of pro-inflammatory cytokines, but the underlying mechanism still needs to be studied.
As already mentioned, adipose tissue has different characteristics and different locations, and visceral adipose tissue has always been shown to be the predominant source of chronic systemic inflammation characteristic of obesity. The conditions associated with insulin resistance, such as obesity and type 2 diabetes, are related to an increase in the circulating concentration of acute phase proteins. The inability to inhibit the production of these proteins by the liver can result in prolonged low-level activation of the immune system, which has been suggested to play a role in the pathogenesis of type 2 diabetes and atherosclerosis [47].
We know that IL-6 is one of the main inflammatory mediators that stimulate the synthesis of acute phase proteins. In addition, some studies have found a directly proportional correlation between the size of the adipocytes with the expression and secretion of IL-6 in vitro, as well as with the interstitial concentrations of IL-6. Although the current findings do not allow an identification of the causal relationships between adipose cell hypertrophy and IL-6 production, reduced gene expression of adiponectin and other adipose differentiation markers, such as aP2 and PPAR-γ, support that IL-6 can exert important paracrine effects on the differentiation of adipose cells [48]. Our in situ results showed that there is a greater immunoreaction for IL-6 in the obese Basal group compared to SupD fed group. These results lead us to the clear question whether this increase is typical of the typical local pro-inflammatory environment that accompanies obesity, and that this is controlled under the administration of the SupD supplement, or else this decrease would be related to an increase in PPAR-γ and the consequent increase in cell differentiation in situ by resident preadipocytes. Because both adipocytes and macrophages are able to secrete a number of biologically active substances, it is important to identify the paracrine signals between adipocytes and macrophages.
The study of immune cells residing in adipose tissue has been carried out for some decades and began with the observation that adipose tissue is an important source of TNF-α and other cytokines such as IL-6, which magnified the effect of overnutrition, also providing a key link between energy metabolism and the immune system [49]. Later, it was shown that many of these cytokines were produced by macrophages in the adipose tissue rather than by the adipocytes themselves, and that these could be observed histologically mainly as part of CLS around adipocytes in necrosis, in the case of obese visceral adipose tissue [50].
We previously argued that our results showed that the non-supplemented obese control (Basal), like that fed with soybean oil, had an important macrophage mononuclear infiltrate, unlike our SupD group that presented less infiltration. However, it was necessary to identify the phenotype of these cells, since the spectrum of infiltrative cells in a tissue can be broad and the functions performed in situ will depend on its type.
M1 or "classically activated" macrophages are induced by proinflammatory mediators such as LPS and IFN-γ. M1 macrophages enhance the production of proinflammatory cytokines (TNF-α, IL-6, IL-12) and generate reactive oxygen species such as NO through the activation of iNOS [55]. M2 macrophages have low expression of pro-inflammatory cytokines and, instead, generate high levels of anti-inflammatory cytokines such as IL-10 [51]. In addition, arginase expression increases in polarized M2 macrophages. This enzyme limits the activity of iNOS through a variety of mechanisms, competing for the arginine substrate that is required for the production of nitric oxide [56]. In general, it is postulated that M2 macrophages participate in the blockade of inflammatory responses and in the promotion of tissue repair; M2 polarization can occur in the absence of IL-4 or IL-13, suggesting that this state can be induced by other regulatory pathways [57].
In addition, recent studies have shown that the IL-4 mediated M2 polarization is associated with activation of transcription factors involved in the oxidative metabolism of lipids, including the coactivator of PPAR-γ and PPAR-β (PGC-1β) [58]. The role of resident macrophages in the development of obesity-induced adipose tissue inflammation, and the obesity-related pathological sequelae including insulin resistance, have been studied intensively [60,61].
It has been demonstrated that macrophages residing in adipose tissue rapidly improve lipid absorption in response to lipolysis of adipocytes, suggesting that macrophages residing in adipose tissue fulfill an adaptive function favoring the uptake of excess FFA, at least during short periods of time. Macrophages are recruited in adipose tissue but, unlike the process of weight loss where lipolysis eventually decreases, chronic stimulation of macrophages recruited into adipose tissue causes local inflammation and an altered metabolic function [59].
An intriguing hypothesis would be that macrophage infiltration can be mechanically linked with increased pathogenicity of intra-abdominal fat [55,56]. It has also been suggested to adipocyte hypertrophy as an underlying mechanism for the recruitment of macrophages, because precipitates necrotic cell death [50,53]. In general terms, apoptosis is not inflammatory, but reflects the packaging of cellular constituents in apoptotic bodies that suppress inflammation. In contrast, during necrosis, the cellular content is released into the extracellular space, where it evokes inflammatory responses [54]. Studies have also shown that macrophages proliferate locally within adipose tissue. In this regard, it has been shown that the amount of macrophages increases significantly in the visceral adipose tissue of ob/ob mice, compared to wild-type mice. This also occurs in subcutaneous adipose tissue but to a lesser degree than visceral adipose tissue. These results confirm that macrophages accumulate mostly in visceral adipose tissue in response to obesity [54].
Other studies have described a "mixed" M1/M2 phenotype for adipose tissue macrophages in obese mice and humans, suggesting that macrophages adopt more complex states in vivo [60,61]. However, the functions of these mixed phenotypes M1/M2 are not completely understood [38]. On the other hand, it has been proposed that during weight gain macrophages undergo a "phenotypic change" from an anti-inflammatory M2 phenotype to a proinflammatory M1 state, a conversion that has been linked to the onset of systemic insulin resistance [63]. It is evident that there is a lot of information that we can gather on this topic.
We decided to perform a phenotypic analysis of macrophage cells focused on visceral adipose tissue, assuming that the tissue is more susceptible to changes caused by diet. Our obese control (Basal) group showed comparatively more cellular infiltrate; the immunoreaction to anti-iNOS monoclonal antibodies, as a molecule characteristic of M1 macrophages, showed that the obese control had the largest population of inflammatory type macrophages and that, as described in the literature, they were mainly surrounding the CLS structures. However, our study group SupD had a lower number of infiltrative macrophage cells, which were more dispersed in the visceral adipose tissue since the number of CLS structures also decreased. Interestingly, our negative control (C-), supplemented with soybean oil, has a high number of mononuclear infiltrative cells but these did not agree with the macrophage phenotype. We found that this group presented an important infiltrate but of lymphocyte type T, since they reacted against pan-lymphocyte antibody CD3. The reasons for this infiltration need to be studied.
Could it be that the incorporation of oil derived from pine seeds would have an effect on the improvement of inflammatory parameters in situ? Probably SupD contains some component that, in some way, signals the improvement of the inflammatory microenvironment, contributing to the change in the phenotype of the resident macrophage cells from a polarization M1 to M2. It was encouraging for us to demonstrate histologically that the visceral adipose tissue in our model supplemented with SupD presented a decrease in infiltrated cells in the adipose tissue and that, in addition, this infiltrate reacted against monoclonal antibodies such as arginase-1 associated with a high immunoreactivity against the anti-inflammatory cytokine IL-10, strongly suggesting the preferential presence of polarized macrophages to M2.
Conclusions
Several studies in animals have shown that dietary supplementation with pine oil, specifically Pinus koraiensis, has beneficial effects in the metabolic context, suggesting that one of its main components, Pinolec acid (PNA), would be responsible for these effects [16,19,20]. The chemical structure of PNA is very similar to its positional isomer gamma-linolenic acid (GLA), which has been shown to have beneficial effects such as decreased inflammation related to some diseases, hypertension and some other chronic diseases [21]. In vitro, it has been shown that PNA could be a potent ligand able to activate dually PPARα/PPARδ [20]. However, there is no current evidence that has demonstrated the presence or tissue enrichment with PNA after oral administration with pine oil.
In summary, our results suggest that in a murine model of diet-induced obesity, dietary supplementation of a high-fat diet with the supplement based on Pinus radiata seed oil, SupD, promotes the hyperplastic growth of small adipocytes, increases vascularity and promotes a local anti-inflammatory environment in visceral TA patches. These observations are in agreement with the systemic metabolic effects observed in vivo, suggesting that the consumption of this product based on Pinus radiata seed oil could be clinically relevant in the control of human obesity.
References
- National health and nutrition examination survey.
- World health organization. Global Status Report on non comunicable diseases (2014).
- Diagnóstico del estado nutricional de menores de 6 años gestantes, nodrizas y adultos ayores, bajo control en el sistema público de salud. Fuente: DEIS (datos: diciembre, (2013).
- http://web.minsal.cl
- Encuensta Nacional de Salud. MINSAL. www.minsal.cl/wp-content/uploads/.../ENS-2016-17_PRIMEROS-RESULTADOS.pdf.
- Effects of dietary restriction on adipose mass and biomarkers of healthy aging in human. Lettieri-Barbato D, Giovannetti E, Aquilano K. 8.12 (2016).
- Cellular and Molecular Players in Adipose Tissue Inflammationin the Development of Obesity-induced Insulin Resistance Byung-Cheol Leea,b and Jongsoon Lee. Biochim biophys acta. 1842.3 (2014): 446-462.
- Christopher X Wong., et al. “Associations of Epicardial, Abdominal, andOverall Adiposity With Atrial Fibrillation”. Circ Arrhythm Electrophysiol 9.12 (2016).
- R Judith Zeller., et al. “Relation Between Obesity, Metabolic Syndrome, Successful Long-Term Weight Reduction, and Right Ventricular Function.” Int Heart J 57 (2016): 441-448.
- Lorraine P Turcotte., et al. “Skeletal Muscle Insulin Resistance: Roles of Fatty Acid Metabolism and Exercise”. Phys Ther 88.11 (2008):1279-1296.
- Thang S Han and Mike EJ Lean. A clinical perspective of obesity, metabolic syndrome andcardiovascular disease. Journal of the Royal Society of Medicine Cardiovascular Diseases 5 (2016): 1-13.
- Kim JK., et al. “Prevention of fat-induced insulin resistance by salicylate”. J Clin Invest 108 (2001): 437-446.
- Sung Sik Choe., et al. “Adipose Tissue Remodeling:its Role in energy Metabolism andMetabolic Disorders.” Frontiers in Endocrinology 7.30 (2016).
- Vincent Zoete a., et al. “Peroxisome proliferator-activated receptor structures: Ligand specificity, molecular switch and interactions with regulators”. Biochimica et Biophysica Acta 1771 (2007): 915-925.
- Christopher Beermann., et al. “Short term effects of dietary medium-chain fatty acids and n-3 long-chain polyunsaturated fatty acids on the fat metabolism of healthy volunteers”. Lipids in Health and Disease (2003).
- Rayalam S., et al. “Resveratrol induces apoptosis and inhibits adipogenesis in 3T3L1 adipocytes”. Phytother Res 22 (2008): 1367-1371.
- Kurimoto Y., et al. “Black soybean seed coat extract ameliorates hyperglycemia and insulin sensitivity via the activation of AMMP- activated protein kinase in diabetic mice”. J Agric Food Chem 23 (2013): 5558-5564
- Wolf RL., et al.“General characteristics of Pinus sp. Seed Fatty acid Compositions, and importance of Δ5-olefinic acids in the taxonomy and phylogeny of the genus”. Lipids 325 (2000): 1-22.
- Le NH., et al. “Diet enriched with korean pine nut oil improves mitochondrial oxidative metabolism in skeletal muscle and brown adipose tissue in diet-induced obesity”. Journal gricol Food Chem 60.48 (2012): 11935-11941.
- Susan Sergeant., et al. “Gamma Linolenic acid, Dihommo gamma linolenic, Eicosanoids and inflamatory Processes”. Eur J Pharmacol 785 (2016): 77-86.
- Wilrike J Pasman., et al. “The effect of Korean pine nut oil on in vitro CCK release, on appetite sensations and on gut hormones in post-menopausal overweight women.” Lipids in Health and Disease (2008).
- Ferramosca A., et al. “Pinus koraiensis seed oil (PinnoThinTM) supplementation reduces body weight gain and lipid concentration in liver and plasma of mice.” Journal of animal and feed sciences 17(2008): 621-630.
- Kirsty L., et al. “Dynamics of Fat Cells Turnover in humans”. Arner. Nature 453.5 (2008).
- M Ikeda, I., et al. “Influence of Korean pine (Pinus koraiensis)-seed oil containing cis-5,cis-9, cis-12-octadecatrienoic acid on polyunsaturated fatty acid metabolism, eicosanoid production and blood pressure of rats”. British Journal of Nutrition 72.5(1994): 775-783.
- Malin Lo¨nn and Kirsten Mehlig. A dipocyte size predicts incidence of type 2 diabetes in women., Calle Bengtsson and Lauren Lissner. The FASEB Journal 24.1 (2016): 326-331.
- Jernås M., et al. “Separation of human adipocytes by size: hypertrophic fat cells display distinct gene expression”. The FASEB Journal 20.9 (2006): 1540-1542.
- Gustafson B and Smith U. “Cytokines Promote Wnt Signaling and Inflammation and Impair the Normal Differentiation and Lipid Accumulation in 3T3-L1 Preadipocytes”. The Journal of Biological Chemistry 281.14 (2006): 9507-9516.
- C Farnier., et al. “Adipocyte functions are modulated by cell size change: potential involvement of an integrin/ERK signalling pathway”. International Journal of Obesity 27.10 (2003): 1178-1186.
- Bernardo Léo Wajchenberg. “Subcutaneous and Visceral Adipose Tissue: Their Relation to the Metabolic Syndrome”. 21.6 Endocrine Reviews (2000): 697-738.
- Corona JC and Duchen MR. “PPARγ as a therapeutic target to rescue mitochondrial function in neurological disease”. J Adipocyte 4.4 (2016): 273-279.
- Weyer C., et al. “Enlarged subcutaneous abdominal adipocyte size, but not obesity itself, predicts type II diabetes independent of insulin resistance”. Diabetologia 43.12 (2000): 1498-506.
- Yang J., et al. “The size of large adipose cells is a predictor of insulin resistance in first-degree relatives of type 2 diabetics”. Obesity (Silver Spring, Md). 20.5 (2012): 932-938.
- T McLaughlin.., et al. “Enhanced proportion of small adipose cells in insulin-resistant vs insulin-sensitive obese individuals implicates impaired adipogenesis”. Diabetologia 50.8 (2007): 1707.
- Choe SS., et al. “Adipose Tissue Remodeling: Its Role in Energy Metabolism and Metabolic Disorders. Frontiers in Endocrinology”. 7 (2016): 30.
- C Farnier., et al. “Adipocyte functions are modulated by cell size change: potential involvement of an integrin/ERK signalling pathway”. International Journal of Obesity 27 (20031178-1186.
- Saverio Cinti., et al. “Adipocyte death defines macrophage localization and function in adipose tissue of obese mice and humans”. The Journal of Lipid Research 46 (2005): 2347-2355.
- Amano SU., et al. “Local proliferation of macrophages contributes to obesity-associated adipose tissue inflammation”. Cell metabolism 19.1 (2014): 162-171.
- Elias I., et al. “Adipose Tissue Overexpression of Vascular Endothelial Growth Factor Protects Against Diet-Induced Obesity and Insulin Resistance”. Diabetes 61.7 (2012): 1801-1813.
- Rutkowski JM., “Mechanisms of obesity and related pathologies: the macro- and microcirculation of adipose tissue”. 276.20 (2009): 5738-5746.
- Justin I Odegaard and Ajay Chawla. “Alternative Macrophage Activation and Metabolism”. Annual Review of Pathology: Mechanisms of Disease 6 (2011): 275-297.
- Chung-Hyun Cho., et al. “Angiogenic Role of LYVE-1–Positive Macrophages in Adipose Tissue”. Circulation Research 100 (2007): e47-e57.
- Satoshi Nishimura., et al. “Adipogenesis in Obesity Requires Close Interplay Between Differentiating Adipocytes, Stromal Cells, and Blood Vessels.” Diabetes 56.6 (2007): 1517-1526.
- Jiro Hirosumi., “A central role for JNK in obesity and insulin resistance”. Nature 420 (2002): 333-336.
- Ansey J., “The Central Role of Perilipin A in Lipid Metabolism and Adipocyte Lipolysis”. IUBMB Life 56 (2004): 379-385.
- Takayoshi Suganami., et al. “A Paracrine Loop between Adipocytes and Macrophages Aggravates Inflammatory Changes. Role of Free Fatty Acids and Tumor Necrosis Factor α”. Arteriosclerosis, Thrombosis, and Vascular Biology 25 (2005): 2062-2068.
- Jean-Philippe Bastard., et al. “Elevated Levels of Interleukin 6 Are Reduced in Serum and Subcutaneous Adipose Tissue of Obese Women after Weight Loss.” The Journal of Clinical Endocrinology & Metabolism 85.9 (2000): 3338-3342.
- Victoria Rotter Sopasakis., et al. “High Local Concentrations and Effects on Differentiation Implicate Interleukin-6 as a Paracrine Regulator. Obesity Research 12.3 (2004): 454-460
- GS Hotamisligil., et al. “Adipose expression of tumor necrosis factor-alpha: direct role in obesity-linked insulin resistance”. Science 259.5091 (1993): 87-91.
- Saverio Cinti., et al. “Adipocyte death defines macrophage localization and function in adipose tissue of obese mice and humans”. The Journal of Lipid Research 46.11 (2005): 2347-235.
- Abul Abbas Andrew H and Lichtman Shiv Pillai. Cellular and Molecular Immunology. 9th Edition.
- AnoopMisra., et al. “Clinical and pathophysiological consequences of abdominal adiposity and abdominal adipose tissue depots”. Nutrition 19.5 (2003): 457-466.
- Ilana Harman-Boehm., et al. “Macrophage Infiltration into Omental Versus Subcutaneous Fat across Different Populations: Effect of Regional Adiposity and the Comorbidities of Obesity.” The Journal of Clinical Endocrinology & Metabolism 92.6 (2007): 2240-2247.
- Fink SL and Cookson BT. “Apoptosis, Pyroptosis, and Necrosis: Mechanistic Description of Dead and Dying Eukaryotic Cells”. Infect Immun 73.4 (2005):1907-1916.
- Lumeng CN., et al. “Obesity induces a phenotypic switch in adipose tissue macrophage polarization”. Journal of Clinical Investigation 117.1 (2007): 175-184.
- Siamon Gordon. “Alternative activation of macrophages”. Nature Reviews Immunology 3.1(2003): 23-35.
- Lamagna C., “Dual role of macrophages in tumor growth and angiogenesis”. Journal of Leukocyte Biology 80.4 (2006): 705-713.
- Divya Vats., et al. “Oxidative metabolism and PGC-1battenuate macrophage-mediated inflammation”. Cell Metabolism 4.1 (2006): 13-24.
- Aliki Kosteli., et al. “Weight loss and lipolysis promote a dynamic immune response in murine adipose tissue”. J Clin Invest 120.10 (2010): 3466-3479.
- Sica A and Mantovani A. “Macrophage plasticity and polarization: in vivo veritas”. The Journal of Clinical Investigation 122.3 (2012):787-795.
- M Zeyda., et al. “Human adipose tissue macrophages are of an anti-inflammatory phenotype but capable of excessive pro-inflammatory mediator production”. International Journal of Obesity 31.9 (2007): 1420-1428.
- Becerra J., et al. “Un producto nutracéutico modulador del apetito y sensibilizador a la Insulina en mamíferos”. Presentación Patente N° 3182-2015. (2015).
- L Weinsier., et al. “The etiology of Obesity: Relative Contribution of Metabolic Factors, Diet, and Physical Activity”. The american Journal of Medicine 105.2 (1998): 145-150.
Citation:
Daniel Duran-Sandoval., et al. “Dietary Supplement Based on Pinus Radiata Seed Oil Modifies the Inflammatory Profile of
Visceral Adipose Tissue in A Murine Model of Diet-Induced Obesity”. Bulletin of Clinical Immunology 1.1 (2018): 50-71.
Copyright: © 2018 Daniel Duran-Sandoval., et al. This is an open-access article distributed under the terms of the Creative Commons Attribution License, which permits unrestricted use, distribution, and reproduction in any medium, provided the original author and source are credited.