Research Article
Volume 1 Issue 2 - 2017
Characterization of A New Bacteriocin Synthesized for the Mexican Bacillus Thuringiensis Strain L4-2 Active Against Listeria Monocytogens and Meticillin-Resistant Staphylococcus Aureus
1Institute of Biotechnology. Faculty of Biological Sciences, Universidad Autónoma de Nuevo León. Pedro de Albay Manuel L. Barragán S/N. University City. San Nicolás de los Garza, N. L. 66450 Mexico
2Laboratory of Conservation Medicine, Center for Genomic Biotechnology. IPN. Blvd. del Maestro S/N esq. Elías Piña, Col. Narcizo Mendoza, Reynosa Tamps. 88710 Mexico
2Laboratory of Conservation Medicine, Center for Genomic Biotechnology. IPN. Blvd. del Maestro S/N esq. Elías Piña, Col. Narcizo Mendoza, Reynosa Tamps. 88710 Mexico
*Corresponding Author: Benito Pereyra-Alférez, 1Institute of Biotechnology. Faculty of Biological Sciences, Universidad Autónoma de Nuevo León. Pedro de Albay Manuel L. Barragán S/N. University City. San Nicolás de los Garza, N. L. 66450 Mexico.
Received: August 18, 2017; Published: September 06, 2017
Abstract
Bacteriocins are proteins with antimicrobial activity that are an alternative for the treatment of foodborne bacteria and fastidious human pathogens. In this work we inspected the antibacterial activity of forty-eight Mexican Bacillus thuringiensis strains against several bacteria used as indicators, such as B. thuringiensis, Listeria monocytogenes (Lm) and a strain of methicillin-resistant Staphylococcus aureus (MRSA). Supernatants from twenty six strains yielded positive action against some indicator, but nine showed inhibitory activity not only for all B. thuringiensis, but also against Lm and MRSA. The strain L4-2, isolated from soil, was selected for microbiological and biochemical studies. The bacteriocin called L4-2 was found in the supernatant from logarithmic growth (4 h) until the stationary phase and decreasing after 8 h. The bacteriocin displayed bacteriolytic and bactericidal activities and had antibacterial action at pH 3.0-7.0 and it remained stable after 30 min at 80°C, but its biological activity was lost after treatment with proteinase K. From Mass spectrometry results showed that the bacteriocin L4-2 possesses a molecular mass of 3,138 Da. The biological activity of bacteriocin L4-2 indicates that it has interesting activity and specificity to be considered for biotechnological purposes.
Keywords: Bacillus thuringiensis; Bacteriocin L4-2; Purification; Bacteriocin
Introduction
Bacteriocins are ribosomally synthesized bacterial polypeptides that develop biological action against very closely related microorganisms or species sharing ecological niches. These molecules have received special interest since those produced by lactic acid bacteria (LAB), especially nisin A, had great results in the biological control of not only foodborne pathogens but also, human infective bacteria (Cherif., et al. 2001; Okuda., et al. 2013). Some special characteristics, for example, i) its proteinaceous nature presupposes easy degradation in the gastric tract in both humans and animals; ii) its high stability and iii) both its broad and narrow spectra of action, establish the bacteriocins as a good candidates for the treatment of some hospital-acquired infections, such as those caused by methicillin-resistant Staphylococcus aureus (MRSA) and Clostridium difficile (Rea., et al. 2010), and also as natural preservatives in foods (Abriouel., et al. 2011; Grande., et al. 2014).
Bacteriocins have been grouped into several classes (Nes., et al. 2007; Cherif., et al. 2003), but according to their structure, they can be divided in two major groups: i) linear and ii) circular peptides. Bacteriocins can or cannot be post-translationally modified and their length varies from 20 to 688 amino acids (Hammami., et al. 2010). Linear bacteriocins include classes I and II, which comprise thermoresistant peptides (lantibiotics) < 5 kDa, and thermo stable peptides <10 kDa with a high glycine content, respectively. Circular bacteriocins have also been classified within the IIc subclass (Grande., et al. 2014), although due to their structure and specificity they could be considered a separate class (Gabrielsen., et al. 2014).
The number and type of bacteriocins is increasing every year, especially those produced by the Bacillus cereus group. This bacterium complex encompasses six species. Among these, Bacillus thuringiensis excels for its capacity to synthesize large proteins with antagonist activity, especially with insecticide and anti-cancer cell activities (Brasseur., et al. 2015). Moreover, this fantastic bacterium could synthesize several proteinaceous metabolites with antibacterial activity. Today, we recognize, at least, 18 peptides from diverse B. thuringiensis strains which display biological action against bacteria and some fungal species (Pacheco-Cano., et al. 2014; Cherif 2003). In this paper we report the screening of B. thuringiensis strains that produce peptides with antimicrobial action. Bacteriocin L4-2, a new bacteriocin produced by soil isolates of B. thuringiensis L4-2 strain, is active against three closely related B. thuringiensis strains and two main fastidious bacteria: L. monocytogenes and a MRSA strain.
Materials and Methods
Bacterial strains
B. thuringiensis strains were obtained from a Mexican entomopathogen bacterium collection that belongs to the Instituto de Biotecnología, FCB-UANL. All B. thuringiensis strains were maintained at −20°C in nutrient broth supplemented with glycerol. Strains used in bioassays as indicators for bacteriocin activity were: i) gram positives: B. thuringiensis HD1, HD73 and GM10 strains; L. monocytogenes and a MRSA strain; ii) gram negatives: Salmonella typhi, Salmonella typhimurium, Salmonella arizonae, Escherichia coli, Klebsiella pneumoniae, and Entrobacter aerogenes. The strains were maintained using tryptic soy broth (TSB) or brain-heart infusion (BHI) culture media.
B. thuringiensis strains were obtained from a Mexican entomopathogen bacterium collection that belongs to the Instituto de Biotecnología, FCB-UANL. All B. thuringiensis strains were maintained at −20°C in nutrient broth supplemented with glycerol. Strains used in bioassays as indicators for bacteriocin activity were: i) gram positives: B. thuringiensis HD1, HD73 and GM10 strains; L. monocytogenes and a MRSA strain; ii) gram negatives: Salmonella typhi, Salmonella typhimurium, Salmonella arizonae, Escherichia coli, Klebsiella pneumoniae, and Entrobacter aerogenes. The strains were maintained using tryptic soy broth (TSB) or brain-heart infusion (BHI) culture media.
Production and bacteriocin bioassays
In order to confirm the presence of the antimicrobial agent in culture, supernatant from forty-eight B. thuringiensis (Table 1) active strains were grown in TSB. In brief, each strain was inoculated in TSB and incubated for 12 h at 28°C in an orbital shaker at 180 rpm. The cultures were centrifuged at 10,000 xg for 30 min at 4°C; supernatants were filtered through 0.45 m pore membranes. Antibacterial activity was detected using the well-diffusion method against the indicator bacterium B. thuringiensis, mentioned in Table 1. Plates were incubated 24 h at 30°C and the diameter of inhibition zone was reported in mm (Jack., et al. 1995; Cherif., et al. 2008) (Table 1). Those strains yielding a halo >10 mm were considered positive, and after that, were tested against MRSA and L. monocytogenes. The supernatant of the positive strains were diluted until activity disappeared (Cherif., et al. 2008). All bioassays were carried out in triplicate and inhibition halo-size was reported as an average.
In order to confirm the presence of the antimicrobial agent in culture, supernatant from forty-eight B. thuringiensis (Table 1) active strains were grown in TSB. In brief, each strain was inoculated in TSB and incubated for 12 h at 28°C in an orbital shaker at 180 rpm. The cultures were centrifuged at 10,000 xg for 30 min at 4°C; supernatants were filtered through 0.45 m pore membranes. Antibacterial activity was detected using the well-diffusion method against the indicator bacterium B. thuringiensis, mentioned in Table 1. Plates were incubated 24 h at 30°C and the diameter of inhibition zone was reported in mm (Jack., et al. 1995; Cherif., et al. 2008) (Table 1). Those strains yielding a halo >10 mm were considered positive, and after that, were tested against MRSA and L. monocytogenes. The supernatant of the positive strains were diluted until activity disappeared (Cherif., et al. 2008). All bioassays were carried out in triplicate and inhibition halo-size was reported as an average.
Time-course of biosynthesis of bacteriocin L4-2
In order to know the growth-phase of maximum bacteriocin production, the B. thuringiensis L4-2 strain was inoculated (10% v/v) in 300 mL Erlenmeyer flasks containing 50 mL of TSB incubated as mentioned before. Samples of 2 mL were removed every 2h. Biomass accumulation was evaluated spectrophotometrically at 600 nm. Each sample was concentrated four times in a SpeedVac concentrator (Savant SVC H110). Antimicrobial activity was evaluated as mentioned before using B. thuringiensis IBGM10 as an indicator. Figure 1 depicts the kinetics of growth and bacteriocin production.
Mode of action of bacteriocin L4-2
The B. thuringiensis IBGM10 strain was used as an indicator in all experiments. This strain was inoculated in 50 mL TSB as mentioned before and at the middle of log phase (4 h); supernatant from the L4-2 strain at 1.2 mg/mL of protein was added. Samples, 1 mL, were taken every hour and biomass accumulation (A600) and viability (CFU mL-1) were recorded. The number of viable cells was evaluated on TSA plates by standard methods.
The B. thuringiensis IBGM10 strain was used as an indicator in all experiments. This strain was inoculated in 50 mL TSB as mentioned before and at the middle of log phase (4 h); supernatant from the L4-2 strain at 1.2 mg/mL of protein was added. Samples, 1 mL, were taken every hour and biomass accumulation (A600) and viability (CFU mL-1) were recorded. The number of viable cells was evaluated on TSA plates by standard methods.
PH, temperature, and proteinase K sensitivity
Partial characterization tests of bacteriocin L4-2 were performed with samples of supernatant from 12 hs of culture. Samples of 500 µL containing 1.2 mg /mL of protein were used to determine sensitivity to temperature, pH and proteinase K. Temperature was evaluated by incubating the samples for 30 min at 40, 50, 60, 70, 80, 90, 100 and 110°C. Supernatant with bacteriocin was incubated for 2 h at 30°C at different pH values using 50 mM citrate buffer (pH 3.0-6.0), 50 mM phosphate buffer (pH 7.0), and 50 mM Tris HCl buffer (pH 8.0-9.0) (Paik., et al. 1997). Buffer without supernatant was used as a control. Sensitivity to proteinase K (1 mg mL-1) was evaluated by incubating samples 1h at 37°C. Proteinase K without bacteriocin was used as a negative control. The effect of bacteriocin L4-2 was assessed by determining the size of the inhibition halo of the B. thuringiensis IBGM10 strain.
Partial characterization tests of bacteriocin L4-2 were performed with samples of supernatant from 12 hs of culture. Samples of 500 µL containing 1.2 mg /mL of protein were used to determine sensitivity to temperature, pH and proteinase K. Temperature was evaluated by incubating the samples for 30 min at 40, 50, 60, 70, 80, 90, 100 and 110°C. Supernatant with bacteriocin was incubated for 2 h at 30°C at different pH values using 50 mM citrate buffer (pH 3.0-6.0), 50 mM phosphate buffer (pH 7.0), and 50 mM Tris HCl buffer (pH 8.0-9.0) (Paik., et al. 1997). Buffer without supernatant was used as a control. Sensitivity to proteinase K (1 mg mL-1) was evaluated by incubating samples 1h at 37°C. Proteinase K without bacteriocin was used as a negative control. The effect of bacteriocin L4-2 was assessed by determining the size of the inhibition halo of the B. thuringiensis IBGM10 strain.
Partial characterization
Starting with a volume of 1.2 L of a 12 h culture at 30°C of the L4-2 strain, the supernatant was filtered on a 0.45 µm membrane and precipitated with 80% ammonium sulfate overnight at 4°C. Precipitated proteins were obtained by centrifugation at 10,000g for 30 min, and were dissolved in 12 mL of Milli-Q water. The sample was passed through FPLC (GE Healthcare, Little Chalfont, UK) using a HiPrep 26/60 Sephacryl S-300 HR column (GE Healthcare) pre-equilibrated and eluted with 50 mM NaH2PO4, pH 7.4 at a flow of 12 mL h-1. Fractions of 5 mL were collected and the protein concentration was evaluated at 280 nm. Antimicrobial activity was evaluated in each fraction. Positive fractions were pooled, lyophilized, and dissolved in 5.0 mL Milli-Q sterile water and passed through filters with different cut-off points, starting with 100 kDa, 30 kDa, 10 kDa and 3 kDa (Centricon® Centrifugal Filter Devices, Merck Millipore, Billerica, MA). The samples obtained in each of the filtration steps were tested with the witness strain, B. thuringiensis IBGM10.
Starting with a volume of 1.2 L of a 12 h culture at 30°C of the L4-2 strain, the supernatant was filtered on a 0.45 µm membrane and precipitated with 80% ammonium sulfate overnight at 4°C. Precipitated proteins were obtained by centrifugation at 10,000g for 30 min, and were dissolved in 12 mL of Milli-Q water. The sample was passed through FPLC (GE Healthcare, Little Chalfont, UK) using a HiPrep 26/60 Sephacryl S-300 HR column (GE Healthcare) pre-equilibrated and eluted with 50 mM NaH2PO4, pH 7.4 at a flow of 12 mL h-1. Fractions of 5 mL were collected and the protein concentration was evaluated at 280 nm. Antimicrobial activity was evaluated in each fraction. Positive fractions were pooled, lyophilized, and dissolved in 5.0 mL Milli-Q sterile water and passed through filters with different cut-off points, starting with 100 kDa, 30 kDa, 10 kDa and 3 kDa (Centricon® Centrifugal Filter Devices, Merck Millipore, Billerica, MA). The samples obtained in each of the filtration steps were tested with the witness strain, B. thuringiensis IBGM10.
Partial purification and molecular mass determination
Two cultures of 3L each were incubated at 28°C for different periods of time; one was incubated for 12h (L4-212) and another for 24h (L4-224). Cells were removed by centrifugation and the supernatants filtered with a 0.45 μm membrane. The filtrates were precipitated with ammonium sulfate as mentioned before. The precipitate was resuspended in 10 mL of sterile Milli-Q water, passed through a 10 kDa cut off filter, and dialyzed using the Mega Dialysis Kit (Sigma-Aldrich, St. Louis, MO) with a molecular weight cut–off of 1000 Da. The L4-212 and L4-224 samples were applied separately to a HPLC system (Agilent 1100 UV System) using a Zorbax 300SB-C18 column (Agilent Technologies Santa Clara, CA) and eluted with a mobile phase: buffer A (90% water: 10% acetonitrile + 0.1% trifluoroacetic acid), and buffer B (10% water and 90% acetonitrile + 0.1% trifluoroacetic acid), at a constant flow of 1.0 mL/min. Absorbance was measured at 280 nm. Peaks were collected, and separately, activity against B. thuringiensis IBGM10 was tested. The bacteriocin obtained in this step was termed L4-2HPLC.
Two cultures of 3L each were incubated at 28°C for different periods of time; one was incubated for 12h (L4-212) and another for 24h (L4-224). Cells were removed by centrifugation and the supernatants filtered with a 0.45 μm membrane. The filtrates were precipitated with ammonium sulfate as mentioned before. The precipitate was resuspended in 10 mL of sterile Milli-Q water, passed through a 10 kDa cut off filter, and dialyzed using the Mega Dialysis Kit (Sigma-Aldrich, St. Louis, MO) with a molecular weight cut–off of 1000 Da. The L4-212 and L4-224 samples were applied separately to a HPLC system (Agilent 1100 UV System) using a Zorbax 300SB-C18 column (Agilent Technologies Santa Clara, CA) and eluted with a mobile phase: buffer A (90% water: 10% acetonitrile + 0.1% trifluoroacetic acid), and buffer B (10% water and 90% acetonitrile + 0.1% trifluoroacetic acid), at a constant flow of 1.0 mL/min. Absorbance was measured at 280 nm. Peaks were collected, and separately, activity against B. thuringiensis IBGM10 was tested. The bacteriocin obtained in this step was termed L4-2HPLC.
The L4-2HPLC sample was applied in an LC-MS system consisting of an EASY-nLC II nanoflow pump (Thermo-Fisher Co. San Jose, CA) coupled to a LTQ Orbitrap Velos mass spectrometer (Thermo-Fisher Co.) with nano-electrospray ionization (ESI). Spectrometer calibration was performed with Calmix® solution containing N-butylamine, caffeine, H2N-MRFA-CO2 and Ultramark 1621. Flow of the LC system was 600 nL/min. The molecular mass of each sample was obtained by processing data using an automatic deconvolution algorithm (Xtract rawfile). The L4-2HPLC sample was processed to determine the amino acid sequence using Collision-Induced Dissociaton (CID) and High Energy Collision Dissociaton (HECD).
Results
Detection and production of bacteriocins
We evaluated the production of bacteriocins as inhibitory substances in 48 native strains of B. thuringiensis using three phylogenetically related strains as indicators. The production of inhibitory substances was demonstrated in the supernatant of 26 strains, of which 24 inhibited the strain HD1 and 8 were specific; while IBGM10 and HD73 were inhibited by 17 and 13, respectively (Table 1). Regarding L. monocytogenes and MRSA, only 9 supernatants had an effect on these two bacteria (Table 1). In all cases, the inhibition zone decreased when the dilution of bacteriocin was increased, suggesting that inhibition was not caused by the activity of a bacteriophage, similar to that reported for entomocin 110 (Cherif., et al. 2008). None of the strains presented an action against the gram negatives tested (data not shown). In this paper, the bacteriocin produced by strain L4-2 was selected for biochemical and bacteriological studies.
We evaluated the production of bacteriocins as inhibitory substances in 48 native strains of B. thuringiensis using three phylogenetically related strains as indicators. The production of inhibitory substances was demonstrated in the supernatant of 26 strains, of which 24 inhibited the strain HD1 and 8 were specific; while IBGM10 and HD73 were inhibited by 17 and 13, respectively (Table 1). Regarding L. monocytogenes and MRSA, only 9 supernatants had an effect on these two bacteria (Table 1). In all cases, the inhibition zone decreased when the dilution of bacteriocin was increased, suggesting that inhibition was not caused by the activity of a bacteriophage, similar to that reported for entomocin 110 (Cherif., et al. 2008). None of the strains presented an action against the gram negatives tested (data not shown). In this paper, the bacteriocin produced by strain L4-2 was selected for biochemical and bacteriological studies.
Bt Strain | Indicator strain* | ||||
HD1 | HD73 | IBGM10 | MRSA | Lm | |
L4-2 | 12 | 19 | 29 | 27 | 29 |
IBGM 11 | 11 | 17 | 26 | 25 | 24 |
IBGM 16 | 11 | 19 | 25 | 25 | 20 |
IBGM 19 | 12 | 21 | 24 | - | - |
IBGM 20 | 10 | - | 22 | - | - |
IBGM 22 | 10 | - | - | - | - |
IBGM 25 | 10 | - | - | - | - |
IBGM 26 | 10 | - | - | - | - |
IBGM 27 | 12 | - | - | - | - |
IBGM 53 | 14 | 17 | 25 | 28 | 25 |
IBGM 57 | 10 | - | 22 | - | - |
IBGM 62 | 12 | 20 | 25 | 26 | 27 |
IBGM 64 | 10 | 18 | 18 | - | - |
IBGM 65 | 10 | 17 | 20 | - | - |
IBGM 67 | 12 | 21 | 25 | 27 | 23 |
IBGM 68 | 10 | 19 | 23 | - | - |
IBGM 70 | 10 | 17 | 24 | - | - |
IBGM 71 | 12 | 21 | 23 | 25 | 27 |
IBGM 72 | 20 | 17 | 25 | 20 | 25 |
IBGM 74 | - | - | 24 | - | - |
IBGM 75 | 20 | - | - | 25 | 28 |
IBGM 76 | - | - | 22 | - | - |
IBGM 81 | 13 | - | - | - | - |
IBGM 82 | 12 | - | - | - | - |
IBGM 83 | 10 | - | - | - | - |
IBGM 85 | 12 | - | - | - | - |
Total | 24 | 13 | 17 | 9 | 9 |
*B. thuringiensis strains HD1, HD73 and IBGM10; MRSA = methicillin-resistant S. aureus; Lm = L. monocytogenes.
Table 1: Inhibitory spectrum of Mexican B. thuringiensis (Bt) strains. Inhibition halo size is represented in mm.
Table 1: Inhibitory spectrum of Mexican B. thuringiensis (Bt) strains. Inhibition halo size is represented in mm.
Synthesis of bacteriocin L4-2 during the growth cycle
The growth kinetics and synthesis of bacteriocin demonstrated that antimicrobial activity began at the mid-log phase, after 4h of incubation, and it decreased starting at 12h, inactivating after 24h (Figure 1). Inactivation may be due to proteolysis of the bacteriocin.
The growth kinetics and synthesis of bacteriocin demonstrated that antimicrobial activity began at the mid-log phase, after 4h of incubation, and it decreased starting at 12h, inactivating after 24h (Figure 1). Inactivation may be due to proteolysis of the bacteriocin.
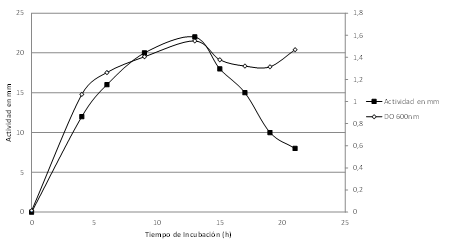
Figure 1: Kinetics of bacteriocin L4-2 production during growth of the L4-2 strain.
Optical density of the culture (◊), inhibitory activity (mm) on the indicator strain (■).
Mode of action
The study of mode of action was approached by incubating bacteriocin with cells of B. thuringiensis IBGM10 as an indicator strain. The indicator strain was grown in STB, and after 4 h of incubation, bacteriocin L4-2 was added. We observed a decrease in the number of viable cells (CFU/mL) (Figure 2A) as well as in optical density (A600) (Figure 2B). This effect remained constant in the following 8 h, suggesting bactericidal and bacteriolytic activity.
The study of mode of action was approached by incubating bacteriocin with cells of B. thuringiensis IBGM10 as an indicator strain. The indicator strain was grown in STB, and after 4 h of incubation, bacteriocin L4-2 was added. We observed a decrease in the number of viable cells (CFU/mL) (Figure 2A) as well as in optical density (A600) (Figure 2B). This effect remained constant in the following 8 h, suggesting bactericidal and bacteriolytic activity.
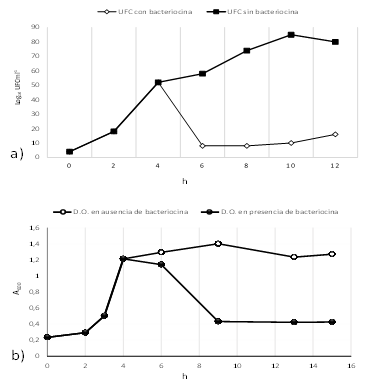
Figure 2: Effect of bacteriocin L4-2 on cell viability. UFC mL-1
(a), and optical density (b) of B. thuringiensis IBGM10.
(a), and optical density (b) of B. thuringiensis IBGM10.
Sensitivity to temperature, pH and proteinase K
The sensitivity of bacteriocin L4-2 to temperature, pH changes and the action of proteinase K was determined by evaluating the residual activity in diffusion assays as well using the B. thuringiensis IBGM10 strain as an indicator. The bacteriocin showed stability even at 80°C, but at 100°C, its inhibitory action was abolished. With regard to pH, bacteriocin activity was present between pH 3.0-7.0. The loss of activity after treatment with proteinase K suggests that bacteriocin L4-2 is proteinaceous.
The sensitivity of bacteriocin L4-2 to temperature, pH changes and the action of proteinase K was determined by evaluating the residual activity in diffusion assays as well using the B. thuringiensis IBGM10 strain as an indicator. The bacteriocin showed stability even at 80°C, but at 100°C, its inhibitory action was abolished. With regard to pH, bacteriocin activity was present between pH 3.0-7.0. The loss of activity after treatment with proteinase K suggests that bacteriocin L4-2 is proteinaceous.
Partial characterization of bacteriocin L4-2
The culture sample of L4-2 concerted 1:100 from its initial volume generated a concentration of 353.89 mg/mL. The sample (12 mL) was fractionated on FPLC into 200 fractions, of which 48 developed inhibition zones against the indicator strain (data not shown). Concentrated and lyophilized samples gave a concentration of 233 mg/mL of protein. The passage of the sample through filters with different cutoffs demonstrated biological activity up to a 10.0 kDa filter. This activity was lost when passed through a 3.0 kDa filter. This suggests that bacteriocin L4-2 must have a size equal or greater than 3.0 kDa.
The culture sample of L4-2 concerted 1:100 from its initial volume generated a concentration of 353.89 mg/mL. The sample (12 mL) was fractionated on FPLC into 200 fractions, of which 48 developed inhibition zones against the indicator strain (data not shown). Concentrated and lyophilized samples gave a concentration of 233 mg/mL of protein. The passage of the sample through filters with different cutoffs demonstrated biological activity up to a 10.0 kDa filter. This activity was lost when passed through a 3.0 kDa filter. This suggests that bacteriocin L4-2 must have a size equal or greater than 3.0 kDa.
Purification of bacteriocin L4-2
HPLC chromatograms of the L4-212 and L4-224 cultures showed different behavior. In L4-212 a peak with a TR 19.929 (Figure 3A) was seen; a finding not seen in L4-224 (Figure 3B). The result of the biological activity of the 19.929 TR fraction showed that it is responsible for the inhibition of the indicator strain; therefore, it is the bacteriocin L4-2 carrier. Mass spectrometry analysis revealed that this molecule has a molecular mass of 3.138 kDa (Figure 4). Results were uncertain in relation to protein sequence, since only a three amino acid (Leu/Leu/Ala) sequence was obtained, even when alternative methods, such as reduction-alkylation, were used. These results suggest that it is a cyclic or compound molecule (Grande., et al. 2014; Gabrielsen., et al. 2014).
HPLC chromatograms of the L4-212 and L4-224 cultures showed different behavior. In L4-212 a peak with a TR 19.929 (Figure 3A) was seen; a finding not seen in L4-224 (Figure 3B). The result of the biological activity of the 19.929 TR fraction showed that it is responsible for the inhibition of the indicator strain; therefore, it is the bacteriocin L4-2 carrier. Mass spectrometry analysis revealed that this molecule has a molecular mass of 3.138 kDa (Figure 4). Results were uncertain in relation to protein sequence, since only a three amino acid (Leu/Leu/Ala) sequence was obtained, even when alternative methods, such as reduction-alkylation, were used. These results suggest that it is a cyclic or compound molecule (Grande., et al. 2014; Gabrielsen., et al. 2014).
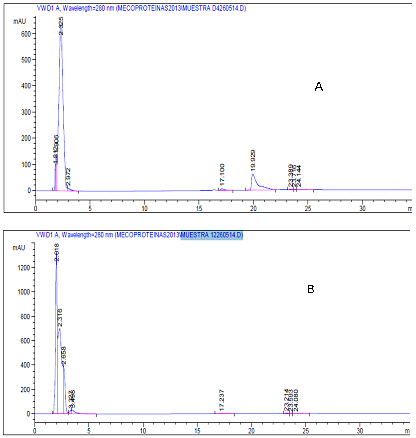
Figure 3: Comparison of the protein file of the supernatant of strain L4-2 cultured for 12h
(A) and 24h (B). Only the 19.929 fraction had inhibitory activity.
Discussion
B. thuringiensis is recognized worldwide for its importance as a biological control agent against insect pests in agricultural crops of importance to the food industry. In addition, B. thuringiensis synthesizes a battery of metabolites with biological activity, including bacteriocins. To date, 18 bacteriocins have been reported synthesized by various strains of B. thuringiensis. These have a high potential for use in the food and pharmaceutical industry (Lopez-Meza., et al. 2015) and in the clinic for control of human pathogens such as Clostridium difficile (Rea., et al. 2010).
In this paper, we searched and selected native strains of B. thuringiensis capable of synthesizing proteins with antimicrobial activity. Of the 48 strains tested, 26 developed inhibition halos against at least one strain of B. thuringiensis used as an indicator. These results with different activity and specificity, suggest that our strains synthesize different bacteriocins, which represent a valuable new reservoir of antimicrobial molecules. Of the 8 strains with a broader spectrum of activity, we selected the L4-2 strain for biochemical and bacteriological studies. This bacteriocin inhibited all the indicator strains, but from the point of view of its action against S. aureus, it is similar to that reported for BLIS (Barboza and Corona 2007) and bacthuricin F4 (Kamoun., et al. 2005); whereas for L. monocytogenes, it resembles thuricin S (Chehimi., et al. 2007), entomocin 110 (Cherif., et al. 2008), entomocin 9 (Cherif., et al. 2003) and thuricin 7 (Cherif., et al. 2001). However, only the L4-2, thuricin (Favret and Younsten, 1989) and thuricin H (Lee., et al. 2009) were active against both pathogens.
Bacteriocin L4-2 production began in the early log phase of the growth cycle as a primary metabolite and continued until the early stationary phase. Most bacteriocin activity occurred at the end of the logarithmic phase, similar to entomocin 110 (Cherif., et al. 2008), thuricin S (Chehimi., et al. 2007), entomocin 9 (Cherif., et al. 2003) and tochicin (Paik., et al. 1997) but activity fell after 12 h of incubation.
Bacteriocin L4-2 showed bactericidal and bacteriolytic effects to the indicator strain, B. thuringiensis IBGM10. Similar results against several strains of B. thuringiensis were found with entomocin 110. This type of effect has been recorded for other bacteriocins such as nisin and Pep5 (Bierbaum and Sahl, 1985; Cherif., et al. 2008). However, in other bacteriocins, such as thuricin 7, bacteriolytic activity was observed only at a higher concentration of the bacteriocin (Cherif., et al. 2001). This type of action shows that the mode of action of bacteriocins may depend on both the concentration of the bacteriocin and the physiological state of the indicator strain (Foulquié Moreno., et al. 2006).
With respect to molecular weight, bacteriocin L4-2 has a mass of 3.138 kDa, similar to thuricin S of 3.137 kDa (Chehimi., et al. 2007) and that of thuricin Bn1 of 3.139 kDa (Ugras., et al. 2013). However, in terms of stability to pH changes, they are very different. Thuricina S is stable at values of 3.0 to 10.5 (Chehimi., et al. 2007) and thuricin Bn1 at 5.0 to 9.0 (Ugras., et al. 2013), while the bacteriocin L4-2 that we report, maintained its activity at pH values of 3.0 to 7.0. The bacteriocin L4-2HPLC was subjected to sequencing experiments, which revealed uncertainty, and we were only able to identify 3 amino acids (Leu/Leu/Ala). This may be because it is a cyclic peptide or compound molecule, as is the case of enterocin AS-48 produced by Enterococcus feacalis (Dimov., et al. 2005; Foulquié Moreno., et al. 2006). Determination of the structure and its amino acid sequence is under development. Our results of molecular mass, characteristic of specificity and mode of action, suggest that the protein L4-2 is a new type of bacteriocin.
Acknowledgements
ELSM was a recipient of a fellowship from the Consejo Nacional de Ciencia y Tecnología (CONACyT), México. MARL is a member of the SNI. This work was supported, in part, by the Project: 20151437 from the Secretaria de Investigación y Posgrado, Instituto Politécnico Nacional.
ELSM was a recipient of a fellowship from the Consejo Nacional de Ciencia y Tecnología (CONACyT), México. MARL is a member of the SNI. This work was supported, in part, by the Project: 20151437 from the Secretaria de Investigación y Posgrado, Instituto Politécnico Nacional.
References
- Abriouel H., et al. “Diversity and applications of Bacillus bacteriocins”. FEMS Microbiology Reviews 35.1(2011): 201-232.
- Bierbaum G and Sahl HG. “Induction of autolysis of staphylococci by the basic peptide antibiotics Pep 5 and nisin and their influence on the activity of autolytic enzymes”. Archives of Microbiology 141.3 (1985): 249-254.
- Brasseur K., et al. “Parasporin-2 from a new Bacillus thuringiensis 4R2 strain induces caspases activation and apoptosis in human cancer cells”. PLoS One 10.8 (2015): e0135106.
- Chehimi S., et al. “Purification and partial amino acid sequence of thuricin S, a new anti-Listeria bacteriocin from Bacillus thuringiensis”. Canadian Journal of Microbiology 53.2 (2007): 284-290.
- Cherif A., et al. “Thuricin 7: a novel bacteriocin produced by Bacillus thuringiensis BMG1.7, a new strain isolated from soil”. Letters in Applied Microbiology 32.4 (2001): 243–247.
- Cherif A., et al. “Detection and characterization of the novel bacteriocin entomocin 9, and safety evaluation of its producer, Bacillus thuringiensis subsp. entomocidus HD9”. Journal of Applied Microbiology95 (2003): 990-1000.
- Cherif A., et al. “Characterization and partial purification of entomocin 110, a newly identified bacteriocin from Bacillus thuringiensis subsp. entomocidus HD110”. Microbiological Research163.6 (2008): 684-692.
- Dimov S., et al. “Bioactive peptides used by bacteria in the concurrence for the ecological niche: general classification and mode of action (overview)”. Biotechnology & Biotechnological Equipment 19 (2005): 3-22.
- Favret ME and Yousten AA. “Thuricin: the bacteriocin produced by Bacillus thuringiensis”. Journal of Invertebrate Pathology 53.2 (1989): 206-216.
- Foulquié Moreno MR., et al. “Isolation and biochemical characterisation of enterocins produced by enterococci from different sources”. Journal of Applied Microbiology 94.2 (2003): 214–229.
- Gabrielsen C., et al. “Circular bacteriocins: biosynthesis and mode of action”. Applied and Environmental Microbiology 80.22 (2014): 6854–6862.
- Grande Burgos MJ., et al., “The cyclic antibacterial peptide enterocin as-48: isolation, mode of action, and possible food applications”. International Journal of Molecular Sciences 15.12 (2014): 22706-22727.
- Hammami R., et al. “BACTIBASE second release: a database and tool platform for bacteriocin characterization”. BMC Microbiology 10.22 (2010): 1-5.
- Jack RW., et al. “Bacteriocins of gram-positive bacteria”. Microbiological Reviews 59.2 (1995):171–200.
- Kamoun F., et al. “Purification, amino acid sequence and characterization of bacthuricin F4, a new bacteriocin produced by Bacillus thuringiensis”. Journal of Applied Microbiology 98.4 (2005): 881-888.
- Lee KD., et al. “The class IId bacteriocin thuricin-17 increases plant growth”. Planta 229.4 (2009): 747-755.
- López-Meza JE., et al. “Antimicrobial Peptides: Current and Potential Applications in Biomedical Therapies”. BioMed Research International (2015).
- Nes IF., et al. “Ribozomally sinthesized antimicrobial peptides (bacteriocin) in lactic acid bacteria: a review”. Food Science and Biotechnology 16 (2007): 657-690.
- Okuda K-I., et al. “Effects of bacteriocins on methicillin-resistant Staphylococcus aureus biofilm”. Antimicrobial Agents and Chemotherapy 57.11 (2013): 5572-5579.
- Paik HD., et al. “Identification and partial characterization of tochicin, a bacteriocin produced by Bacillus thuringiensis subsp. tochigiensis". Journal of Industrial Microbiology and Biotechnology 19.4 (1997): 294-298.
- Pacheco-Cano RD., et al. “Characterization, N-terminal sequencing and classification of Tolworthcin 524: A bacteriocin produced by Bacillus thuringiensis subsp. tolworthi". Microbiological Research 169.12 (2014): 948–953.
- Rea M., et al. “Classification of bacteriocins from Gram-positive bacteria”. Prokaryotic antimicrobial peptides: From Genes to Applications (2011): 29–53.
- Riley MA and Wertz JE. “Bacteriocins: Evolution, ecology, and application”. Annual Review of Microbiology 56 (2002): 117-137.
- Ugras S., et al. “Purification and characterization of the bacteriocin Thuricin Bn1 produced by Bacillus thuringiensis subsp. kurstaki Bn1 Isolated from a Hazelnut Pest”. Journal of Microbiology and Biotechnology 23.2 (2013): 167–176.
Citation:
Benito Pereyra-Alférez., et al. “Characterization of A New Bacteriocin Synthesized for the Mexican Bacillus Thuringiensis Strain L4-2 Active Against Listeria Monocytogens and Meticillin-Resistant Staphylococcus Aureus”. Clinical Biotechnology and Microbiology 1.2 (2017): 70-79.
Copyright: © 2017 Benito Pereyra-Alférez., et al. This is an open-access article distributed under the terms of the Creative Commons Attribution License, which permits unrestricted use, distribution, and reproduction in any medium, provided the original author and source are credited.