Review Article
Volume 2 Issue 1 - 2018
Biogenic– Biosynthesis Metallic Nanoparticles (MNPs) for Pharmacological, Biomedical and Environmental Nanobiotechnological Applications
Chemistry Department, Faculty of Science and Arts, Baljarashi, Al Baha University, Baljarashi 65635, Saudi Arabia
*Corresponding Author: Loutfy H Madkour, Chemistry Department, Faculty of Science and Arts, Baljarashi, Al Baha University, Baljarashi 65635, Saudi Arabia.
Received: January 04, 2018; Published: January 31, 2018
Abstract
In future, the biogenic– biosynthesis MNPs have wide perspective synthesis in healthcare, sustainable and renewable energy and other commercial products. MNPs produced by nanotechnology have received global attention due to their extensive applications in the biomedical and physiochemical fields. Biomolecules present in live plants, plant extracts and microorganisms such as: bacteria, fungi, seaweeds, actinomycetes, algae and microalgae can be used to reduce metal ions to MNPs in a single-step and green synthesis process. Biological green synthesis of MNPs has been always beneficial, more economical, energy efficient and eco-friendly approach, which is free of toxic contaminates as required in therapeutic applications. The biosynthesis reduction of metal ion to base metal is quite rapid, readily conducted at room temperature, pressure and easily scaled up.
The reducing agents involved include the various water-soluble plant metabolites (e.g. alkaloids, phenolic compounds, terpenoids, flavonoids, saponins, steroids, tannins and other nutritional compounds) and co-enzymes. The polysaccharides, proteins and lipids present in the algal membranes act as capping agents and thus limit the use of non-biodegradable commercial surfactants, which are difficult to remove after the synthesis of MNPs. Metallic nanoparticles viz. cobalt, copper, silver, gold, platinum, zirconium, palladium, iron, cadmium and metal oxides such as titanium oxide, zinc oxide, magnetite, etc. have been the particular focus of green biosynthesis. Here we review the methods of making MNPs using plants extracts and microorganisms. Methods of particle characterization, biomedical and environmental applications of MNPs are reviewed. In the near future, the application of clean, non-toxic, and eco-friendly nanostructured material will be possible in industry and biomedicine.
Keywords: Biogenic–Biosynthesis; MNPs; Plant extracts; Microorganisms; Pharmacological; Biomedical; Commercial applications
Introduction
Nanobiotechnology, bionanotechnology, and nanobiology are terms that have emerged in reference to the combination of nanotechnology and biology. Through the convergence of these disciplines, the production of metallic nanoparticles (MNPs) using biological material as reducing agents is rapidly progressing. Nanotechnology has become one of the most important technologies in all areas of science. It relies on the synthesis and modulation of nanoparticles, which requires significant modifications of the properties of metals [1]. Nanomaterials have in fact been used unknowingly for thousands of years; for example, gold nanoparticles that were used to stain drinking glasses also cured certain diseases. Scientists have been progressively able to observe the shape- and size-dependent physiochemical properties of nanoparticles by using advanced techniques. Recently, the diverse applications of metal nanoparticles have been explored in biomedical, agricultural, environmental, and physiochemical areas (Figure1) [1–5].
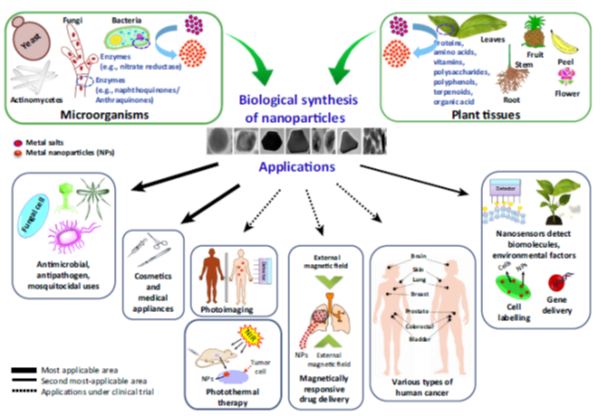
Figure 1: Biological Synthesis and Applications of Metal Nanoparticles in Biomedical and Environmental Fields.
The field of nanotechnology has proved to be one of the most active areas of research [6-14], mainly encompasses with biology, physics, chemistry and material sciences and it develops novel therapeutic nanosized materials for biomedical and pharmaceutical applications.
A Japanese researcher, Norio Taniguchi, first introduced Nanotechnology [15]. Over time, the application of this science became common in various fields such as material sciences, electronics, and biotechnology [16-19]. Nanoparticles are of great scientific interest as they bridge the gap between bulk materials and atomic or molecular structures [20]. Among various nanoparticles, metal nanoparticles are the most promising ones and this is due to their anti-bacterial properties which, occurs because of the high surface to volume ratio. Change in the size or surface of the composition can change the physical and chemical properties of the nanoparticles [21,22]. In recent decades, the application of metal nanoparticles is very common due to their wide applications in various industries [23].
By reaching nanoparticles size in a certain range (1–100 nm), their physical, chemical and electrical properties will change. These properties depend on silver nanoparticles size and characteristics such as melting temperature, magnetic behavior, redox potential and their color can be controlled by changing their size and shapes [24]. In recent years silver nanoparticles have attracted a lot of attentions due to their good conductivity, chemical stability, use as catalysts [25] and their applications in various industries including the medical sciences, in order to deal with HIV virus, food industries as anti-bacterial agents in food packing [26], anti-bacterial properties [27] and also their unique electrical and optical qualities [28,29].
Pinning down a definition of nanotechnology
Nanotechnology is a rapidly growing field of science, which is particularly interesting for researchers since the early 90s of the last century. This area has become an integral part of modern technology [30]. Nanotechnology is said to be a “key technology of the 21st century”, which is the result of its interdisciplinary nature [31]. Because nanotechnology is still evolving, there doesn’t seem to be any one definition that everybody agrees on. We know that nano deals with matter on a very small scale - larger than atoms and molecules, but smaller than a breadcrumb. We know that matter at the nano scale can behave differently than bulk matter. Beyond that, different individuals and groups focus on different aspects of nanotechnology as a discipline. Here are a few definitions of what nanotechnology is for your consideration.
Nanotechnology is a rapidly growing field of science, which is particularly interesting for researchers since the early 90s of the last century. This area has become an integral part of modern technology [30]. Nanotechnology is said to be a “key technology of the 21st century”, which is the result of its interdisciplinary nature [31]. Because nanotechnology is still evolving, there doesn’t seem to be any one definition that everybody agrees on. We know that nano deals with matter on a very small scale - larger than atoms and molecules, but smaller than a breadcrumb. We know that matter at the nano scale can behave differently than bulk matter. Beyond that, different individuals and groups focus on different aspects of nanotechnology as a discipline. Here are a few definitions of what nanotechnology is for your consideration.
- Nanotechnology is the study and use of structures between 1 nanometer (nm) and 100 nanometers in size.
- This is probably the most barebones and generally agreed upon definition of nanotechnology. To put these measurements in perspective, compare your one meter (about three feet three inches) high hall table to a nanometer. You would have to stack one billion nanometer-sized particles on top of each other to reach the height of your hall table. Another popular comparison is that you can fit about 80,000 nanometers in the width of a single human hair.
- The word nano is a scientific prefix that stands for 10-9 or one-billionth; the word itself comes from the Greek word nanos, meaning dwarf.
- “Structures, devices, and systems having novel properties and functions due to the arrangement of their atoms on the 1 to 100 nanometer scale. Many fields of endeavor contribute to nanotechnology, including molecular physics, materials science, chemistry, biology, computer science, electrical engineering, and mechanical engineering.”
- This definition from The Foresight Institute adds a mention of the various fields of science that come into play with nanotechnology.
- “Nanotechnology is the study of phenomena and fine-tuning of materials at atomic, molecular and macromolecular scales, where properties differ significantly from those at a larger scale. Products based on nanotechnology are already in use and analysts expect markets to grow by hundreds of billions of euros during this decade.” The European Commission offers this definition of what nanotechnology is, which both repeats the fact mentioned in the previous definition that materials at the nanoscale have novel properties, and positions nano vis-a-vis its potential in the economic marketplace.
- “Nanotechnology is the understanding and control of matter at dimensions between approximately 1 and 100 nanometers, where unique phenomena enable novel applications. Encompassing nanoscale science, engineering, and technology, nanotechnology involves imaging, measuring, modeling, and manipulating matter at this length scale.”
- This definition from the National Nanotechnology Initiative adds the fact that nanotechnology involves certain activities, such as measuring and manipulating nanoscale matter.
- “[Nanotechnology is] an upcoming economic, business, and social phenomenon. Nano-advocates argue it will revolutionize the way we live, work and communicate.” This last is taken from a definition of nanotechnology by Thomas Thesis, director of physical sciences at the IBM Watson Research Center. It offers a broader and interesting perspective of the role and value of nanotechnology in our world.(Excerpted from Nanotechnology for Dummies (2nd edition), from Wiley Publishing).
The Nanotechnology Debate
Nanotechnology deals with the production and usage of material with nanoscale dimension. There are many different points of view about the nanotechnology. These differences start with the definition of nanotechnology. Some define it as any activity that involves manipulating materials between one nanometer and 100 nanometers. However the original definition of nanotechnology involved building machines at the molecular scale and involves the manipulation of materials on an atomic (about two-tenths of a nanometer) scale.
Nanotechnology deals with the production and usage of material with nanoscale dimension. There are many different points of view about the nanotechnology. These differences start with the definition of nanotechnology. Some define it as any activity that involves manipulating materials between one nanometer and 100 nanometers. However the original definition of nanotechnology involved building machines at the molecular scale and involves the manipulation of materials on an atomic (about two-tenths of a nanometer) scale.
The debate continues with varying opinions about exactly what nanotechnology can achieve. Some researchers believe nanotechnology can be used to significantly extend the human lifespan or produce replicator-like devices that can create almost anything from simple raw materials. Others see nanotechnology only as a tool to help us do what we do now, but faster or better.
The third major area of debate concerns the timeframe of nanotechnology-related advances. Will nanotechnology have a significant impact on our day-to-day lives in a decade or two, or will many of these promised advances take considerably longer to become realities? Finally, all the opinions about what nanotechnology can help us achieve echo with ethical challenges. If nanotechnology helps us to increase our lifespans or produce manufactured goods from inexpensive raw materials, what is the moral imperative about making such technology available to all? Is there sufficient understanding or regulation of nanotech based materials to minimize possible harm to us or our environment? Only time will tell how nanotechnology will affect our lives.
Nanotechnology Applications
The ability to see nano-sized materials has opened up a world of possibilities in a variety of industries and scientific endeavors. Because nanotechnology is essentially a set of techniques that allow manipulation of properties at a very small scale, it can have many applications, such as the ones listed below.
The ability to see nano-sized materials has opened up a world of possibilities in a variety of industries and scientific endeavors. Because nanotechnology is essentially a set of techniques that allow manipulation of properties at a very small scale, it can have many applications, such as the ones listed below.
Drug delivery (medicine.html). Today, most harmful side effects of treatments such as chemotherapy are a result of drug delivery methods that don't pinpoint their intended target cells accurately. Researchers at Harvard and MIT [32] (http://www.rsc.org/chemistryworld/News/2006/April/11040601.asp) have been able to attach special RNA strands, measuring about 10 nm in diameter, to nanoparticles and fill the nanoparticles with a chemotherapy drug. These RNA strands are attracted to cancer cells. When the nanoparticle encounters a cancer cell it adheres to it and releases the drug into the cancer cell. This directed method of drug delivery has great potential for treating cancer patients while producing less side harmful effects than those produced by conventional chemotherapy.
Fabrics (fabrics.html). The properties of familiar materials are being changed by manufacturers who are adding nanosized components to conventional materials to improve performance. For example, some clothing manufacturers are making water and stain repellent clothing using nano-sized whiskers (http://www.sciencentral.com/articles/view.php3?article_id=218392126&language=english) in the fabric that cause water to bead up on the surface.
Reactivity of Materials. The properties of many conventional materials change when formed as nano-sized particles (nanoparticles). This is generally because nanoparticles have a greater surface area per weight than larger particles; they are therefore more reactive to some other molecules. For example studies have shown that nanoparticles of iron can be effective in the cleanup of chemicals in groundwater (nanoparicles-iron-water-pollution-cleanup.html) because they react more efficiently to those chemicals than larger iron particles. Strength of Materials. Nano-sized particles of carbon, (for example nanotubes and bucky balls) are extremely strong.
Nanotubes and Bucky balls are composed of only carbon and their strength comes from special characteristics of the bonds between carbon atoms. One proposed application that illustrates the strength of nanosized particles of carbon is the manufacture of t-shirt weight bullet proof vests made out of carbon nanotubes (http://www.nanowerk.com/spotlight/spotid=1054.php) [33]. Micro/Nano Electromechanically Systems (mems.html). The ability to create gears, mirrors, sensor elements, as well as electronic circuitry in silicon surfaces allows the manufacture of miniature sensors such as those used to activate the airbags in your car. This technique is called MEMS (Micro-Electromechanically Systems). The MEMS technique results in close integration of the mechanical mechanism with the necessary electronic circuit on a single silicon chip, similar to the method used to produce computer chips. Using MEMS to produce a device reduces both the cost and size of the product, compared to similar devices made with conventional methods. MEMS is a stepping stone to NEMS or Nano-Electro Mechanical Systems. NEMS products are being made by a few companies, and will take over as the standard once manufacturers make the investment in the equipment needed to produce nano-sized features.
Molecular Manufacturing (molecular-manufacturing.html). If you're a Star Trek fan, you remember the replicator, a device that could produce anything from a space age guitar to a cup of Earl Grey tea. Your favorite character just programmed the replicator, and whatever he or she wanted appeared. Researchers are working on developing a method called molecular manufacturing that may someday make the Star Trek replicator a reality. The gadget these folks envision is called a molecular fabricator; this device would use tiny manipulators to position atoms and molecules to build an object as complex as a desktop computer. Researchers believe that raw materials can be used to reproduce almost any inanimate object using this method.
Nanotechnology is rapidly growing by producing nanoproducts and nanoparticles (NPs) that can have novel and size-related physico-chemical properties differing significantly from larger matter [34]. The novel properties of NPs have been exploited in a wide range of potential applications in medicine, cosmetics, renewable energies, environmental remediation and biomedical devices [35–37]. Nanoscience is a new interdisciplinary subject that depends on the fundamental properties of nanosize objects [38,39].
Nanoparticles possess wondrous optical, electronic, magnetic, and catalytic properties than the bulk material owing to their high surface area to volume ratio [40,41]. Metal nanoparticles like silver and gold show different colors due to their Surface Plasmon Resonance (SPR) phenomenon. It is a collective oscillation of free electrons of the metal nanoparticles in resonance with the frequency of the light wave interactions causing the SPR band to appear in the visible and infrared region [42].
Nanotechnology is an important field of modern research dealing with synthesis, strategy and manipulation of particle’s structure ranging from approximately 1 to 100 nm in size. Within this size range all the properties (chemical, physical and biological) changes in fundamental ways of both individual atoms/molecules and their corresponding bulk. Novel applications of nanoparticles and nanomaterials are growing rapidly on various fronts due to their completely new or enhanced properties based on size, their distribution and morphology. It is swiftly gaining renovation in a large number of fields such as health care, cosmetics, biomedical, food and feed, drug-gene delivery, environment, health, mechanics, optics, chemical industries, electronics, space industries, energy science, catalysis, light emitters, single electron transistors, nonlinear optical devices and photo-electrochemical applications. Tremendous growth in these expanding technologies had opened applied frontiers and novel fundamentals.
Nanoparticles NPs and their applications
In the past decade there has been a marked increase in the field of fabrication of nanoparticles with controlled morphologies and remarkable features making it an extensive area of research. The synthesis of nanoparticles (NPs) with control over particle size, shape and crystalline nature has been one of the main objectives in chemistry that could be used for potential applications, such as bio-medical, biosensor, catalyst for bacterial biotoxin elimination and lower cost electrode [43-45].
In the past decade there has been a marked increase in the field of fabrication of nanoparticles with controlled morphologies and remarkable features making it an extensive area of research. The synthesis of nanoparticles (NPs) with control over particle size, shape and crystalline nature has been one of the main objectives in chemistry that could be used for potential applications, such as bio-medical, biosensor, catalyst for bacterial biotoxin elimination and lower cost electrode [43-45].
The nanoparticles (NPs) having at least one dimension less than 100 nm such as nanosheets, nanotubes and nanowires have gained much attention because of their promising applications [46-48]. Other than their unique physical and chemical properties, NPs act as a bridge between bulk materials and atomic or molecular structures. Therefore, they are good candidate for applications including medical, catalysis, electrochemistry, biotechnology, and trace-substance detection [49-52].
In other words, we can say that nanoparticles are the collection of atoms bonded together with a structural radius of less than 100 nm. A nanoparticle is the most essential component in the creation of a nanostructure, and is far smaller than the world of everyday objects that are described by Newton’s laws of motion, but bigger than an atom or a simple molecule that are governed by quantum mechanics.
Nanoparticles are very ordinary in nature - for instance, proteins exist in almost all biological Systems. These can include, e.g., fullerenes, metal clusters, large molecules, such as proteins, and even hydrogen-bonded assemblies of water molecules which exist in water at ambient temperatures. Nanomaterials are increasingly becoming a part of our daily lives. They are characterized by new properties that do not accompany to their counterparts existing at the macro scale. Therefore, nanomaterials are used in innovative products and processes [53]. According to the recommendations of the European Commission 2011/696/EU, nanoparticles (also called nanocrystals or nanopowders) are defined as “natural, randomly created or manufactured materials containing particles in a free state or as and aggregate or agglomerate, wherein at least 50% of the particles has one or more dimensions in the range 1−100 nm. In certain cases, justified on grounds of environmental protection, health, safety or competitiveness, one can take a value between 1−50 percent”.
The recommendation 2011/696/EU also states that: “Where technically feasible and requested in specific legislation, compliance with definition may be determined on the basis of the specific surface area by volume. A material falls under the definition if its specific surface area by volume is greater than 60 m2 cm-3” [54].The vast majority of manufactured nanomaterials are available in different shapes and sizes. It is expected that their use will significantly increase in the next decade. Currently, nanomaterials are produced in the hundreds of thousands of tons. They are used in a variety of products, including consumer electronics, automobiles, aerospace, sporting goods, household, hygiene, construction and medical. According to a statement issued by the European Commission, the global amount of manufactured nanomaterials is close to 11.5 million of tones, which is equivalent to their market value reaching 20 billion per year (this estimate also applies to soot and amorphous silica). It is estimated that the current global market for nanomaterials is from 300 000 tons up to 1.6 million tons. The Asian region accounts for largest market share (approx. 34%), followed by North America (approx. 31%) and Europe (approx. 30%). Table 1 includes market for nanomaterials - global production in 2014 [53].
Production | Nanoparticles | Minimum global production volume [tons] | Maximum global production volume [tons] |
High volume production | Titanium dioxide | 60,000 | 150,00 |
Zinc oxide | 32,000 | 36,00 | |
Silicon dioxide | 185,000 | 1,400,000 | |
Aluminium oxide | 5,000 | 10,100 | |
Carbon Nanotubes |
1,550 | 1,950 | |
Nanoclays | 25,000 | 51,000 | |
Cerium oxide | 880 | 1,400 | |
Low volume production | Quantum dots | 4.5 | 9 |
Antimony tin oxide | 120 | 225 | |
Copper oxide | 290 | 570 | |
Silver | 135 | 420 | |
Nanocellulose | 400 | 1,350 | |
Bismuth oxide | 35 | 55 | |
Cobalt oxide | 5 | <10 | |
Dendrimers | 0.3 | 1.25 | |
Fullerenes & POSS | 40 | 100 | |
Graphene | 60 | 80 | |
Gold | 1 | 3 | |
Iron oxide | 9 | 45 | |
Magnesium oxide | 15 | 30 | |
Manganese oxide | 2 | 3.5 | |
Nickel | 5 | 20 | |
Zirconium oxide | 80 | 300 |
Table 1: Market for nanomaterials - global production in 2014 [53].
According to the definition, NPs occur naturally or can be prepared intentionally. The latter group is divided into the following categories [53]:
- Non-metallic inorganic nanoparticles (TiO2, SiO2, ZnO, Al (OH)3, Fe2O3, Fe3O4, CeO2, ZrO2, CaO, ITO, ATO)
- Metals and metal alloys (Au, Ag, Pt, Pd, Cu, Fe, Ni, Co, Al, Mn, Mo)
- Nanomaterials based on carbon (fullerenes, carbon nanotubes, carbon nanofibers, graphene)
- Nanopolymers and dendrimers (polymeric nanoparticles, polymer nanotubes, nanowires and nanorods, nanocellulose, nanostructured polymer films)
- Quantum dots (cadmium telluride, cadmium selenide, quantum dots free of cadmium).
Production of nanomaterials may be carried out by three types of methods:
- Biological (production of nanoparticles by microorganisms),
- Chemical (e.g. chemical vapour deposition CVD, chemical reduction),
- Physical (e.g. physical vapour deposition, PVD, production of thin films).
Nanoparticle has multifunctional properties and very interesting applications in various fields such as medicine, nutrition and energy [55]. Figure 2 shows the general applications of metal nanoparticles in biological field.
The biogenic syntheses of monodispersed nanoparticles with specific sizes and shapes have been a challenge in biomaterial science. Also, it has created remarkable advantages in the pharmacological industry to cure various bacterial and viral diseases [56]. Biosynthesis methods have more compensation over other classical synthesis procedures due to the availability of more biological entities and eco-friendly procedures. The rich biodiversity and easy availability of plant entities have been highly explored for the nanomaterials synthesis [57]. Recently, the biosynthesis of nanosized particles, wires, flowers, tubes was reported successfully. These biological synthesized nanomaterials have potential applications in different areas such as treatment, diagnosis, development surgical nanodevices and commercial product manufacturing [58]. Nanomedicine makes a huge impact in healthcare sector in treating various chronic diseases. Hence, eco-friendly synthesis of nanoparticles is considered as building blocks of the forthcoming generations to control various diseases [59]. Plant crude extract contains novel secondary metabolites such as phenolic acid, flavonoids, alkaloids and terpenoids in which these compounds are mainly responsible for the reduction of ionic into bulk metallic nanoparticles formation [60]. These primary and secondary metabolites are constantly involved in the redox reaction to synthesize eco-friendly nanosized particles.
Many previous reports are demonstrating that biosynthesized nanoparticle effectively controlled oxidative stress, genotoxicity and apoptosis related changes [61]. Additionally, nanoparticles have broad application in agriculture industry and plant sciences. For instance, the nanoparticle using bioprocessing technology converts the agricultural and food wastes into energy and useful by-products. Based on that, the review focused on biosynthesized metallic nanoparticles from plant derivatives and its application in medical and commercial sectors including waste water treatment, cosmetics and food industry.
Types of Nanoparticles (NPs)
Elements: You will find an extensive range of element nanopowders under one roof that is Mk nano. We have aluminum, boron, carbon, cobalt, iron, gold, tin, titanium, etc.you name it, and we have it.
Elements: You will find an extensive range of element nanopowders under one roof that is Mk nano. We have aluminum, boron, carbon, cobalt, iron, gold, tin, titanium, etc.you name it, and we have it.
Gold: Gold nanoparticles (AuNPs) are used in immunochemical studies for identification of protein interactions. They are used as lab tracer in DNA fingerprinting to detect presence of DNA in a sample. They are also used for detection of aminoglycoside antibiotics like streptomycin, gentamycin and neomycin. Gold nanorods are used to detect cancer stem cells, beneficial for cancer diagnosis and for identification of different classes of bacteria.
Silver: Silver nanoparticles are the most effective amongst all because of its good antimicrobial efficacy against bacteria, viruses and other eukaryotic microorganisms. They are unquestionably the most widely used nanomaterials. They are used as antimicrobial agents in textile industries for water treatments.
Alloys:We offer the finest quality of alloy nanopowders at a single click. Some of the alloy nanopowders offered here are copper- tin, iron-cobalt, silver-copper and copper zinc.
Single element oxides:An oxide is a chemical compound that contains at least one oxygen atom and one other element in its chemical formula. Metal oxides contain an anion of oxygen in the oxidation state of -2. We offer aluminum hydroxide nanopowder, aluminum oxide hydroxide nanopowder, aluminum oxide nanopowder, antimony oxide nanopowder, bismuth oxide nanopowder, boron oxide nanopowder and many more. Alloy nanoparticles displays structural properties that are different from their bulk samples. Since Ag i.e. silver has the highest electrical conductivity among metal fillers and, unlike many other metals, their oxides have relatively better conductivity.
Multi element oxides:Multiple oxides have two nonequivalent metal sites and have amazing properties resulting in extensive application in various fields. Some of the multi element oxide offered by Mk nano is aluminum cerium oxide nanopowder, ATO-antimony tin oxide nanopowder, barium strontium titanium oxide nanopowder, calcium titanium oxide nanopowder, titanium silicate nanopowder and so on.
Carbides, nitrides and other compounds: Carbon compounded with a non-metal such as calcium, silicon, boron or metals like cobalt, titanium, tungsten, or vanadium. They are characterized by their extreme hardness and resistance to high temperatures. They are used as abrasives, in cutting, drilling, grinding, and polishing tools.
A nitride is a compound of nitrogen where nitrogen has a formal oxidation state of -3. Nitrides are a large class of compounds with various properties and a wide range of applications. We have a wide range of carbides, nitrides and other such compounds available at one place.
Mesoporous materials: A mesoporous material is a material containing pores with diameters ranging between 2 and 50 nm. Porous materials are classified based on their sizes. Check the amazing range of mesoporous materials under the nanoparticles tab. We at Mk Nano offer you the finest quality of nanoparticles at the best price. We have a range of elements, alloys, single element oxide, multi element oxides, carbides, nitrides and other compounds, mesoporous materials.
Magnetic: Magnetic nanoparticles are those, which can be manipulated using magnetic field gradients. Such particles commonly consists of magnetic elements such as iron, nickel and cobalt and their chemical compounds. They are actively investigated for targeted cancer treatment (magnetic hyperthermia), stem cell sorting and manipulation, guided drug delivery, gene therapy, DNA analysis, and magnetic resonance imaging. The nanoparticles have diameters less than 1 micrometer, the larger microbeads diameter ranges from 0.5-500 micrometer.
Several individual magnetic nanoparticles together form a magnetic nanoparticle cluster that are termed as magnetic nano beads their diameter ranges from 50- 200 nanometers. Magnetic nanoparticles have been a very popular topic in the field of research due to its amazing properties. They can be used in catalysis, in biomedicine, magnetic resonance imaging, magnetically tunable colloidal photonic crystals, microfluidics, data storage, environmental remediation, nanofluids, optical filters and defect sensor.
Types of Magnetic Nanoparticles
Ferrites
Ferrite nanoparticles are the iron oxides in the crystal structure of maghemite or magnetite. They are the most explored magnetic nanoparticles till date. They become super magnetic when the ferrite particles become lesser than 128 nm which prevent self-agglomeration, because they display their magnetic behaviours only when an external magnetic field is applied.
Ferrites
Ferrite nanoparticles are the iron oxides in the crystal structure of maghemite or magnetite. They are the most explored magnetic nanoparticles till date. They become super magnetic when the ferrite particles become lesser than 128 nm which prevent self-agglomeration, because they display their magnetic behaviours only when an external magnetic field is applied.
Ferrites with a Shell
The surface of a magnetite magnetic nanoparticle is inert and usually resists covalent bonds with functionalization molecules. Though, the reactivity can be enhanced by coating a layer of silica on the surface. The silica shell can be easily altered with numerous surface functional groups. Apart from this, some fluorescent dye molecules can also be covalently bonded to the functionalized silica shell. Some advantage of Ferrite nanoparticle clusters coated with a silica shell over metallic nanoparticles are:
The surface of a magnetite magnetic nanoparticle is inert and usually resists covalent bonds with functionalization molecules. Though, the reactivity can be enhanced by coating a layer of silica on the surface. The silica shell can be easily altered with numerous surface functional groups. Apart from this, some fluorescent dye molecules can also be covalently bonded to the functionalized silica shell. Some advantage of Ferrite nanoparticle clusters coated with a silica shell over metallic nanoparticles are:
- Advanced chemical stability
- Narrow size distribution
- Developed colloidal constancy
- Magnetic moment are tuned with the nanoparticle cluster size
- Retained super paramagnetic properties
- Silica surface allows direct covalent functionalization
Metallic
These nanoparticles have multiple uses in technical areas due to their higher magnetic moment whereas oxides would be beneficial for biomedical applications. There are also certain disadvantages associated with them, they are pyrophoric and reactive to oxidizing agents to various degrees that makes their management tough. It allows unwanted side reactions which decreases their suitability for biomedical applications.
Metallic with a Shell
The metallic core of a magnetic nanoparticle can be passivated by oxidation, surfactants, polymers and precious metals. Nanoparticles with a magnetic core consisting either of elementary iron or cobalt with a nonreactive shell made of graphene have been produced. Its advantages compared to ferrite nanoparticles are listed below:
The metallic core of a magnetic nanoparticle can be passivated by oxidation, surfactants, polymers and precious metals. Nanoparticles with a magnetic core consisting either of elementary iron or cobalt with a nonreactive shell made of graphene have been produced. Its advantages compared to ferrite nanoparticles are listed below:
- Developed magnetization
- Greater stability in acidic, basic solution as well as in organic solvents
Properties of Magnetic Nanoparticles
Physical Properties
Magnetic effects are produced by activities of particles that have both mass and electric charges. A rotating, electric-charged particle generates a magnetic dipole termed as magneton. In ferromagnetic materials, magnetons are connected in groups. A magnetic domain refers to a volume of ferromagnetic material in which all magnetons are aligned in the same direction by the exchange forces.
Physical Properties
Magnetic effects are produced by activities of particles that have both mass and electric charges. A rotating, electric-charged particle generates a magnetic dipole termed as magneton. In ferromagnetic materials, magnetons are connected in groups. A magnetic domain refers to a volume of ferromagnetic material in which all magnetons are aligned in the same direction by the exchange forces.
Magnetic Properties
Materials are classified by their reaction to an externally applied magnetic field. Descriptions of orientations of the magnetic moments in a material aid in finding diverse forms of magnetism. The basic types if magnetism can be classified into diamagnetism, paramagnetism, ferromagnetism, antiferromagnetism, and ferrimagnetism. When there is an external applied magnetic field, the atomic current loops formed by the orbital motion of electrons respond to oppose the applied field.
Materials are classified by their reaction to an externally applied magnetic field. Descriptions of orientations of the magnetic moments in a material aid in finding diverse forms of magnetism. The basic types if magnetism can be classified into diamagnetism, paramagnetism, ferromagnetism, antiferromagnetism, and ferrimagnetism. When there is an external applied magnetic field, the atomic current loops formed by the orbital motion of electrons respond to oppose the applied field.
Synthesis of Magnetic Nanoparticle
Some of the most established methods of synthesis of magnetic nanoparticle are:
Some of the most established methods of synthesis of magnetic nanoparticle are:
- Co-precipitation - It is a convenient way to synthesize iron oxides from aqueous Fe2+/Fe3+ salt solutions. It is done by adding a base under inert atmosphere at room temperature or at raised temperatures. The size, shape, and composition of the magnetic nanoparticles depends on the type of salts used. This method is widely used to produce ferrite nanoparticles of controlled sizes and magnetic properties.
- Thermal Decomposition - The smaller sized magnetic nanocrystals can be synthesized by thermal decomposition of organometallic compounds in high-boiling organic solvents containing stabilizing surfactants.
- Microemulsion - With this method, platinum alloys, and gold-coated platinum nanoparticles have been manufactured in reverse micelles of cetyltrimethlyammonium bromide.
- Flame spray synthesis - With the use of flame spray pyrolysis and changing the reaction conditions, oxides, metal or carbon coated nanoparticles are produced at a rate of > 30 g/h.
Applications of Magnetic Nanoparticles
They have usage in many different fields, few of them are listed below
They have usage in many different fields, few of them are listed below
Medical Field
Magnetic nanoparticles are used in an experimental cancer treatment called magnetic hyperthermia. Magnetic nanoparticles are used for the detection of cancer. They are conjugated with carbohydrates and used for detection of bacteria.
Magnetic nanoparticles are used in an experimental cancer treatment called magnetic hyperthermia. Magnetic nanoparticles are used for the detection of cancer. They are conjugated with carbohydrates and used for detection of bacteria.
Treatment of Wastewater
They have a good potential for treatments of contaminated water. The amazing property of easy separation by applying a magnetic field and the large surface area makes this possible.
They have a good potential for treatments of contaminated water. The amazing property of easy separation by applying a magnetic field and the large surface area makes this possible.
Chemistry
They can be used as a catalyst or catalyst supports in chemical reactions. The support may be inert or they may participate in the catalytic reactions. Magnetic iron oxide nanocrystals, monodisperse magnetic cobalt nanoparticles and ferrite magnetic nanopowders have many industrial applications.
They can be used as a catalyst or catalyst supports in chemical reactions. The support may be inert or they may participate in the catalytic reactions. Magnetic iron oxide nanocrystals, monodisperse magnetic cobalt nanoparticles and ferrite magnetic nanopowders have many industrial applications.
Genetic Engineering
They can be used for a whole lot of genetics applications. One such application is the isolation of mRNA.
They can be used for a whole lot of genetics applications. One such application is the isolation of mRNA.
Characterization and Properties of nanoparticles (MNPs)
After green synthesis of NP, characterization is an important step to identify NP by their shape, size, surface area and dispersity [62]. A homogeneity of these properties is important in many applications. For this purpose, various characterization techniques have been developed as analytical tools (Figure 3).The common techniques of characterizing nanoparticles are as follows: UV–visible spectrophotometry, dynamic light scattering (DLS), scanning electron microscopy (SEM), transmission electron microscopy (TEM), Fourier transform infrared spectroscopy (FTIR), powder X-ray diffraction (XRD) and energy dispersive spectroscopy (EDS) [63-65].
After green synthesis of NP, characterization is an important step to identify NP by their shape, size, surface area and dispersity [62]. A homogeneity of these properties is important in many applications. For this purpose, various characterization techniques have been developed as analytical tools (Figure 3).The common techniques of characterizing nanoparticles are as follows: UV–visible spectrophotometry, dynamic light scattering (DLS), scanning electron microscopy (SEM), transmission electron microscopy (TEM), Fourier transform infrared spectroscopy (FTIR), powder X-ray diffraction (XRD) and energy dispersive spectroscopy (EDS) [63-65].
The UV–visible spectroscopy is a commonly used techniques [66]. Light wavelengths in the 300–800 nm are generally used for characterizing various metal nanoparticles in the size range of 2 to 100 nm [63]. Spectrophotometric absorption measurements in the wavelength ranges of 400–450 nm [67] and 500–550 nm [68] are used in characterizing the silver and gold nanoparticles, respectively. The dynamic light scattering (DLS) is used to characterize the surface charge and the size distribution of the particles suspended in a liquid [62].
Electron microscopy is another commonly used method of characterization [69]. Scanning electron microscopy and transmission electron microscopy are used for morphological characterization at the nanometer to micrometer scale [70]. The transmission electron microscopy has a 1000-fold higher resolution compared with the scanning electron microscopy [71]. FTIR spectroscopy is useful for characterizing the surface chemistry [72]. Organic functional groups (e.g. carbonyls, hydroxyls) attached to the surface of nanoparticles and the other surface chemical residues are detected using FTIR.
XRD is used for the phase identification and characterization of the crystal structure of the nanoparticles [73]. X-rays penetrate into the nanomaterial and the resulting diffraction pattern is compared with standards to obtain structural information. Elemental composition of metal nanoparticles is commonly established using energy dispersive spectroscopy (EDS) [74]. Auger electron microscopy (AEM), scanning probe electron microscopy (SPM), X-ray photo electron microscopy (XPS), time of flight-secondary ion mass spectroscopy (TOF-SIMS) for primary surface analysis; low energy ion scattering (LEIS) for identification of elements present in the outer most surface of the material under examination; scanning tunneling microscopy (STM), atomic force microscopy (AFM) for surface characterization at atomic scale; inductively coupled plasma-optical emission spectroscopy (ICP-OES) for optical properties; surface enhanced Raman scattering (SERS) for single molecular attachments to the surface of NP.
The properties and behaviour of materials at the nanoscale differ significantly when compared to microscale. The nanoparticles show enhanced electrical, optical and magnetic properties. There are two basic factors which cause that nanomaterials behave differently than macromaterials. These are surface effects (properties of surface atoms fraction) and quantum effects. These factors affect the chemical reactivity of materials, as well as they determine their mechanical, optical, electrical and magnetic properties [75].
Compared to microparticles, the fraction of surface atoms in nanoparticle is increased. In relation to microparticles, nanoparticles are characterized by increased mass of surface particles. The ratio of surface area to mass in nanometric particles is 1000-fold greater than in micrometric particles [53]. The nanometric particles are thus characterized by increased chemical reactivity, which is approximately 1000-fold higher compared to micromaterials. Quantum dots are the materials which in greatest extent use the quantum effects [76].
Their electron behaviour is similar to the behaviour of single atoms or small molecules. Quantum dots are described as artificial atoms. Another result of the quantization effect is the appearance of magnetic moments in nanoparticles, while they do not occur in bulk materials such as gold, platinum or palladium. Magnetic moments are due to the presence of several unpaired electron spins formed by a few hundred atoms. Quantum effects also affect the ability to accept or donate electrical charge, which determines the catalytic ability [53].
In addition to potential benefits (antibiotic, antifungal and antiviral properties) [77], silver nanoparticles (AgNPs) can trigger for instance undesirable and hazardous interactions with biological systems, thereby generating toxicity [78-80].
Physical properties
Their properties can be majorly divided into physical and optical properties:
Their properties can be majorly divided into physical and optical properties:
- They have a large surface area
- Nanoparticles of yellow gold and gray silicon are red in colour
- Zinc oxide particles have been found to have superior UV blocking properties compared to its bulk substitute
- Gold nanoparticles melt at much lower temperatures (~300 °C for 2.5 nm size) than the gold slabs (1064 °C)
- Absorption of solar radiation in photovoltaic cells is much higher in nanoparticles than it is in thin films of continuous sheets of bulk material - since the particles are smaller, they absorb greater amount of solar radiation.
Optical properties
Nano particles also often possess unexpected optical properties, as they are small enough to confine their electrons and produce quantum effects. One example of this is that gold nanoparticles appear deep red to black in solution.
Nano particles also often possess unexpected optical properties, as they are small enough to confine their electrons and produce quantum effects. One example of this is that gold nanoparticles appear deep red to black in solution.
Magnetization and other properties
Other properties unique among nanoparticles are quantum confinement in semiconductor particles, surface Plasmon resonance in some metal particles and superparamagnetism in magnetic materials.
Other properties unique among nanoparticles are quantum confinement in semiconductor particles, surface Plasmon resonance in some metal particles and superparamagnetism in magnetic materials.
Diffusion properties
At elevated temperatures especially, nanoparticles possess the property of diffusion.
At elevated temperatures especially, nanoparticles possess the property of diffusion.
Metallic Nanoparticle Synthesis Using Plants
Biosynthesis of metal nanoparticles using plant derivatives is extremely studied in the last two decades. Different synthetic methods have been employed for the preparation of MNPs with diverse morphology and size. Although these methods have resulted in superior MNPs but still a key understanding of improved manufacturing process is required which could be exploited at the industrial and commercial level to have better built, long lasting, cleaner, safer and smarter products such as home appliances, communication technology, medicines, transportation, agriculture and industries.
Biosynthesis of metal nanoparticles using plant derivatives is extremely studied in the last two decades. Different synthetic methods have been employed for the preparation of MNPs with diverse morphology and size. Although these methods have resulted in superior MNPs but still a key understanding of improved manufacturing process is required which could be exploited at the industrial and commercial level to have better built, long lasting, cleaner, safer and smarter products such as home appliances, communication technology, medicines, transportation, agriculture and industries.
Therefore, the main focus is to design MNPs using environmentally benign approaches. These provide solutions to growing challenges related to environmental issues. The non-biological methods (chemical and physical) are used in the synthesis of nanoparticles, which has a serious hazardous and high toxicity for living organisms. Recently, many studies have proved that the plant extracts act as a potential precursor for the synthesis of nanomaterial NPs in non-hazardous ways. In addition, the biological synthesis of metallic nanoparticles is inexpensive, single step and eco-friendly methods. Different macro–microscopic organisms such as plant, bacteria, fungi, seaweeds and microalgae are carrying out the biological syntheses of nanoparticles. The biosynthesized nanomaterials have been effectively controlling the various endemic diseases with less adverse effect. Synthesis of nanoparticles is increasing exponentially because of its wide range of applications in the field of optoelectronics, biosensors, bio-nanotechnology, biomedicine etc. [81-87]. Various physical and chemical methods have been formulated for the synthesis of nanopartilces of desired shape and size. However these methods are not economically feasible and environment friendly. Therefore, green synthesis has been considered as one of the promising method for synthesis of nanopartilces because of their biocompatibility, low toxicityand eco-friendly nature [88]. Various microorganism and plants have proved to be a source of inspiration fornanomaterial synthesis. Some well-known examples of nanoparticles synthesized by microorganisms either intracellularly [89] or extracellularly [90] are: synthesis of magnetite by magnetotactic bacteria [91-93] and synthesis of siliceous material by radiolarians and diatoms [94-96].
A mixture of curiosity and unshakable belief that mother earth has developed the best method for the synthesis of nano range of materials have led to a new and exciting field of research which involves microorganism and plants for the synthesis of nanomaterials. The green synthesis methods include synthesis of nanoparticles using microorganisms like bacteria, fungus, yeasts [97], plants [98-100] and DNA [101]. Multiple species of bacteria and fungi have been investigated for the growth of nanoparticles of different composition and size, for example, synthesis of gold by Verticilliumsp [97], synthesis of CdS quantum dots using fungi etc. [102].
Besides microbes, use of part of plants like stem, leaves, roots etc. [98] for the synthesis of nanoparticles is yet another exciting possibility that is relatively unexplored. Advantage of using plants over microorganism is the elimination of the elaborate process of cell culture. Moreover, nanoparticles synthesized using biological methods are more compatible for medical use as compared to chemical and physical methods where toxic material may adsorb on the surface of the nanoparticles that may have adverse effect when used for medicinal purpose. The biosynthesis method employing plant extracts of Pelargonium graveolens, Medicagosativa, Azadirachta indica, Lemongrass, Aploevera, Cinnamomum Camphor [68, 98-100] have drawn great attention as an alternative to conventional methods, because plants are found in abundance in nature.
Dimensionality of nanoparticles
They are generally classified based on their dimensionality, morphology, composition, uniformity, and agglomeration.
They are generally classified based on their dimensionality, morphology, composition, uniformity, and agglomeration.
1. Dnanomaterials:These are one dimensional in the nanometer scale are typically thin films or surface coatings, and include the circuitry of computer chips and the antireflection and hard coatings on eyeglasses. These have been used in electronics, chemistry, and engineering.
2. Dnanomaterials:Two-dimensional nanomaterials have two dimensions in the nanometer scale. These include 2D nanostructured films, with nanostructures firmly attached to a substrate, or nanopore filters used for small particle separation and filtration. Asbestos fibers are an example of 2D nanoparticles.
3. Dnanomaterials:Materials that are nanoscaled in all three dimensions are considered 3D nanomaterials. These include thin films deposited under conditions that generate atomic-scale porosity, colloids, and free nanoparticles with various morphologies.
Structural transformation in monolayer materials: a 2D to 1D transformation
Reducing the dimensions of materials to atomic scales results in a large portion of atoms being at or near the surface, with lower bond order and thus higher energy. At such scales, reduction of the surface energy and surface stresses can be the driving force for the formation of new low-dimensional nanostructures, and may be exhibited through surface relaxation and/or surface reconstruction, which can be utilized for tailoring the properties and phase transformation of nanomaterials without applying any external load. Kasra et al., [103] used atomistic simulations and revealed an intrinsic structural transformation in monolayer materials that lowers their dimension from 2D nanosheets to 1D nanostructures to reduce their surface and elastic energies (Figure 4).
Reducing the dimensions of materials to atomic scales results in a large portion of atoms being at or near the surface, with lower bond order and thus higher energy. At such scales, reduction of the surface energy and surface stresses can be the driving force for the formation of new low-dimensional nanostructures, and may be exhibited through surface relaxation and/or surface reconstruction, which can be utilized for tailoring the properties and phase transformation of nanomaterials without applying any external load. Kasra et al., [103] used atomistic simulations and revealed an intrinsic structural transformation in monolayer materials that lowers their dimension from 2D nanosheets to 1D nanostructures to reduce their surface and elastic energies (Figure 4).
Experimental evidence of such transformation has also been revealed for one of the predicted nanostructures. Such transformation plays an important role in bi-/multi-layer 2D materials. Several methods are used for synthesis of nanoparticles (NPs) such as physical, chemical, enzymatic and biological. Physical methods are including plasma arcing, ball milling, thermal evaporate, spray pyrolysis, ultra-thin films, pulsed laser desorption, lithographic techniques, sputter deposition, layer by layer growth, molecular beam epistaxis and diffusion flame synthesis of nanoparticles [104].
Similarly, the chemical methods are used to synthesized NPs by electrodeposition, sol–gel process, chemical solution deposition, chemical vapour deposition [105,106], soft chemical method, Langmuir Blodgett method, catalytic route, hydrolysis (Pileni, 1997), co-precipitation method and wet chemical method [107]. Physical and chemical methods have been using high radiation and highly concentrated reductants and stabilizing agents that are harmful to environmental and to human health.
Hence, biological synthesis of nanoparticles is a single step bioreduction method and less energy is used to synthesize eco-friendly NPs [108]. Apart from that, the biological methods are using eco-friendly resources such as plant extracts, bacteria, and fungi, micro algae such as cyanobacteria, diatom, seaweed (macroalgae) and enzymes [109]. Figure 5 shows different types of metallic nanoparticles synthesized from plant resources. Nature has provided ways and insight into the synthesis of advanced nanomaterials.
It has now been reported in the literature that biological systems can act as the ‘bio-laboratory’ for the production of pure metal and metal oxide particles at the nanometer scale using biomimetic approach. Various microorganisms, such as bacteria [110,111], fungi [112,113], yeast [114], plant extracts [115] and waste materials [116], have acted as eco-friendly precursors for the synthesis of NPs with potential applications. The biological approach which includes different types of microorganisms has been used to synthesize different metallic MNPs, which has advantages over other chemical methods as this is greener, energy saving and cost effective. The coating of biological molecules on the surface of MNPs makes them biocompatible in comparison with the MNPs prepared by chemical methods [117-119].
The biocompatibility of bio-inspired NPs offers very interesting applications in biomedicine and related fields [120]. The biogenic methods lead to the designing of NPs with interesting morphologies and varied sizes [121,122]; for example, Ag NPs in the size range of 25 ± 12 nm have been prepared by exposing fungal biomass (Verticillium) to the aqueous solution of Ag+ ions, where the NPs were not toxic since the biomass (fungal cells) continues to grow. The MNPs were found to grow on the surface of mycelia as a result of electrostatic interaction between the Ag+ ions and negatively charged carboxylate groups of enzymes present in the cell wall of the fungus [118]. Pt NPs of definite shape and size have been prepared from the cell-soluble protein extract of sulfate reducing bacteria [121].
These MNPs fabricated via biogenic enzymatic process were superior to those synthesized via chemical methods as the use of expensive chemicals was limited and they possessed higher catalytic activity. An industrially important fungus, Penicillium rugulosum, was used to synthesize uniform sized Au NPs, which is easier to handle as compared to other bacteria and yeast [123]. Extracts from plant leaf, root, latex, seed and stem have also been used for the synthesis of MNPs as they act as stabilizing or reducing agents. The leaf extracts of Jasminum sambac were employed to prepare stable Au, Ag, Au–Ag alloy NPs [124]. Regarding the morphology control, triangular, hexagonal and spherical shaped Au NPs were prepared using hot water, olive leaf extract at a high reaction temperature as compared to MNPs synthesized via chemical methods [125]. Iron–polyphenol (Fe–P) NPs with a photocatalytic activity against Acid Black-194 dye were synthesized from Australian native leaves of Eucalyptus tereticornis, Melaleuca nesophila and Rosemarinus officinalis [126]. It was noted that the polyphenols present in the three plants reacted with ferric chloride (FeCl3) solution to form chelated ferric–polyphenols NPs and also led to different shapes of Fe–P NPs.
Among different biological systems used for NP synthesis, various forms of algae are now being currently used as model systems as these have tremendous ability of bioremediation of toxic metals thereby converting them into more pliable forms. Also, these are competent in the fabrication of diverse metal and metal oxide NPs [127]. The biosynthesis of MNPs using algae and waste materials is an emerging and upcoming research. Figure 6 represents green synthesis of MNPs using algae and waste materials.
Bio-reduction mechanism
The methods for making nanoparticles can generally involve either a “top down” approach or a“bottom up” approach [128]. In top-down synthesis (Figure 7), nanoparticles are produced by size reduction from a suitable starting material [129]. Size reduction is achieved by various physical and chemical treatments (Figure 7). Top down production methods introduce imperfections in the surface structure of the product and this is a major limitation because the surface chemistry and the other physical properties of nanoparticles are highly dependent on the surface structure [130]. In bottom up synthesis, the nanoparticles are built from smaller entities, for example by joining atoms, molecules and smaller particles [118]. In bottom up synthesis, the nanostructured building blocks of the nanoparticles are formed first and then assembled to produce the final particle [130]. The bottom up synthesis mostly relies on chemical and biological methods of production.
The methods for making nanoparticles can generally involve either a “top down” approach or a“bottom up” approach [128]. In top-down synthesis (Figure 7), nanoparticles are produced by size reduction from a suitable starting material [129]. Size reduction is achieved by various physical and chemical treatments (Figure 7). Top down production methods introduce imperfections in the surface structure of the product and this is a major limitation because the surface chemistry and the other physical properties of nanoparticles are highly dependent on the surface structure [130]. In bottom up synthesis, the nanoparticles are built from smaller entities, for example by joining atoms, molecules and smaller particles [118]. In bottom up synthesis, the nanostructured building blocks of the nanoparticles are formed first and then assembled to produce the final particle [130]. The bottom up synthesis mostly relies on chemical and biological methods of production.
The probable mechanism of nanoparticle synthesis by bottom up approach is shown in Figure 8.
Of the biological methods of synthesis, the methods based on microorganisms have been widely reported [131-139]. Microbial synthesis is of course readily scalable, environmentally benign and compatible with the use of the product for medical applications, but production of microorganisms is often more expensive than the production of plant extracts. Plant mediated nanoparticle synthesis using whole plant extract or by living plant were also reported in literature [140,141].
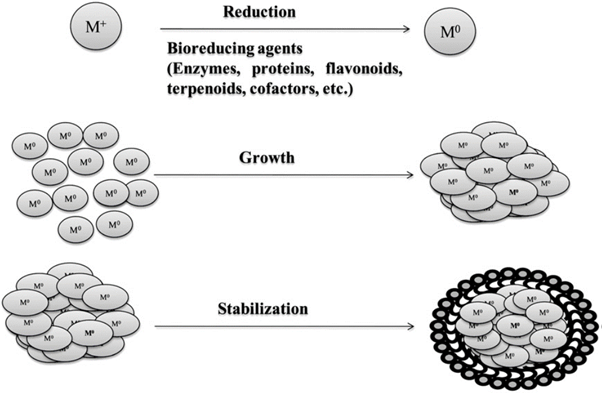
Figure 9: Possible chemical constituents of plant extract responsible for the bioreduction of metal ions [142,143].
Silver: the biochemical reaction of AgNO3 reacts with plant broth leads to the formation of AgNPs by following reaction [144]. Figure 9. [142,145], shows the probable chemical constituents present in the plant extract responsible for the bioreduction of metal ions, their growth and stabilization.
Figure 10 explains the proposed mechanism of biological synthesis of nanoparticles.
Ag+ NO-3 + Plant extract → Ag° NPs + byproducts
Gold: The plant extract contains different biomolecules such as proteins, sugars, amino acids, enzymes and other traces of metals. These metabolites are strongly involved in the bio-reduction process. The proposed reaction was Au+ ions reduction into metallic Auo nanoparticles in the presence of metabolites and redox enzymes [130]. The reaction is given below.
H Au+ Cl4. 4H2O + Plant extracts → Au°NPs + byproducts
Platinum: Platinum is involved in the following reduction process such as H2 Pt+ Cl2. 6H2O + Plant extracts → Pt°NPs + byproducts
Copper: The copper nanoparticles are synthesized from plant extracts and the reduction mechanism was proposed by [145]: CuSO4. 5H2O + Plant metabolites → Cu°NPs + byproducts
Zinc oxide: A typical procedure was employed in ZnO nanoparticles production, the zinc nitrate was dissolved in the aloe plant extract to produce the nanosized particles. The method is as follows [146]: Zinc nitrate + Plant extract → ZnO + byproducts
Titanium oxide
Nano-sized TiO2 has been successfully produced by adding TiO (OH)2 solution to the suspension of Lactobacillus sp. [147]. The equation can be described as following: Ti. (OH)2 → TiO2 + H2O
Nano-sized TiO2 has been successfully produced by adding TiO (OH)2 solution to the suspension of Lactobacillus sp. [147]. The equation can be described as following: Ti. (OH)2 → TiO2 + H2O
It was concluded that the oxido-reductase generated on the cell surface results in the reaction occurrence. However, so far, there is still no clear and thorough understanding on the synthesis of nanoparticles by microbes. Self-defense is the essential action to prevent from hazard for each single life in the ecosystem, which has been survived after natural selection. Microbes will release specific enzymes, which is capable of removing toxic ions, to protect themselves while the danger is detected.
The enzyme will disperse on the cell surface and in the solution. As the ions meet the enzyme, the reaction to form compound nanoparticles will occur and precipitate on the cell surfaces and in the solutions. The nanoparticles were found on the cells but not in the solution because enzymes barely spread to solution much due to the stickiness of cytolemma, and also the enzyme may have been involved into nanoparticle synthesis reactions before it could diffuse into the solutions, or detection of nanoparticles in the solution has not been paid enough attention. Briefly, it is mainly suspected that the electrostatic interaction and specific enzyme(s) of microbes are significant factors in the formation of nanoparticles, even though the real mechanism of biosynthesis of nanoparticles is still unclear.
Different parts of plants used to produce metallic MNPs
In producing nanoparticles using plant extracts, the extract is simply mixed with a solution of the metal salt at room temperature. The reaction is complete within minutes. Nanoparticles of silver, gold and many other metals have been produced this way [135]. Figure 11 shows picture of various plants used for the biosynthesis of nanoparticles. The nature of the plant extract, its concentration, the concentration of
In producing nanoparticles using plant extracts, the extract is simply mixed with a solution of the metal salt at room temperature. The reaction is complete within minutes. Nanoparticles of silver, gold and many other metals have been produced this way [135]. Figure 11 shows picture of various plants used for the biosynthesis of nanoparticles. The nature of the plant extract, its concentration, the concentration of
The metal salt, the pH, temperature and contact time are known to affect the rate of production of the nanoparticles, their quantity and other characteristics [148]. Recently, the plant mediated nanomaterial has drawn more attention due to its vast application in various fields due to their physic-chemical properties. The different metallic nanoparticles such as gold, silver, platinum, zinc, copper, titanium oxide, magnetite and nickel were synthesized from natural resources and have been studied exclusively. The different parts of plant such as stem, root, fruit, seed, callus, peel, leaves and flower are used to syntheses of metallic nanoparticles in various shapes and sizes by biological approaches. Figure 12 shows different sizes and shapes of the nanoparticles derived from plants resources. Biosynthesis reaction can be altered by wide range of metal concentration and amount of plant extract in the reaction medium, it may transform the shapes and size of the nanoparticles [149-151].
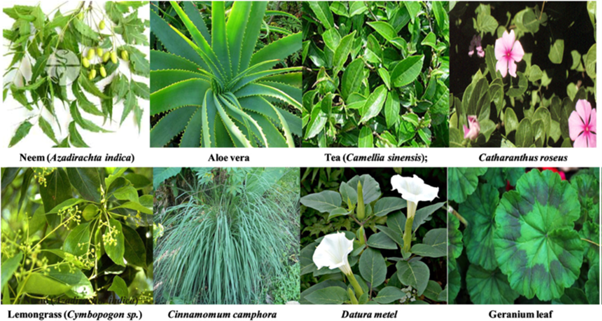
Figure 12: Different size and shapes of the nanoparticle synthesis from plant extracts (derived from [149-151]).
Stem as source
The stem part of plant extract shows the different functional groups, particularly the carboxyl, amine, and phenolic compounds that are involved in the reduction of metal ions. Some previous studies [152] are proposed model mechanisms of nanoparticles interaction with pathogenic organisms. Therefore, the biosynthesized metal nanoparticles acted as good antibacterial agents.
The stem part of plant extract shows the different functional groups, particularly the carboxyl, amine, and phenolic compounds that are involved in the reduction of metal ions. Some previous studies [152] are proposed model mechanisms of nanoparticles interaction with pathogenic organisms. Therefore, the biosynthesized metal nanoparticles acted as good antibacterial agents.
Fruits mediated synthesis
The extract contains active phytochemical compounds that are liable for the single step reduction reaction. The use of optimum physic chemical parameters to synthesize nanomaterial is very effective in pharmacological solicitation to treat various endemic diseases.
The extract contains active phytochemical compounds that are liable for the single step reduction reaction. The use of optimum physic chemical parameters to synthesize nanomaterial is very effective in pharmacological solicitation to treat various endemic diseases.
Seeds as source
The fenugreek seed extract contains high flavonoids and other natural bioactive products such as lignin, saponin and vitamins. The reduction of chloroauric acid by using the powerful reducing agents fenugreek seed extract acts as a better surfactant. The COO- group (carboxylic), C = N and C = C functional groups are present in the seed extract. The functional group of metabolites acts as a surfactant of gold nanoparticles and the flavonoids can stabilize the electrostatic stabilization of gold NPs [100]. The aqueous extract of Macrotyloma uniflorum enhanced the reduction rate of some metal ions. This may be owing to the presence of caffeic acid in the extract. Therefore, the presence of caffeic acid reduction reaction was occurred within a minute.
The fenugreek seed extract contains high flavonoids and other natural bioactive products such as lignin, saponin and vitamins. The reduction of chloroauric acid by using the powerful reducing agents fenugreek seed extract acts as a better surfactant. The COO- group (carboxylic), C = N and C = C functional groups are present in the seed extract. The functional group of metabolites acts as a surfactant of gold nanoparticles and the flavonoids can stabilize the electrostatic stabilization of gold NPs [100]. The aqueous extract of Macrotyloma uniflorum enhanced the reduction rate of some metal ions. This may be owing to the presence of caffeic acid in the extract. Therefore, the presence of caffeic acid reduction reaction was occurred within a minute.
Leaves mediated synthesis
Plant leaves extract used as a mediator to synthesis of nanoparticles was reported. Leaves of Centella asiatica, Murraya koenigii, Alternanthera sessilis and many plants leaves extract have been studied [152]. Recently, P. nigrum leaves were stated to contain an important bioactive compound, which is involved in the nanoparticle synthesis by eco-friendly method. It shows a significant tool for antimicrobial agents in present and in a near future.
Plant leaves extract used as a mediator to synthesis of nanoparticles was reported. Leaves of Centella asiatica, Murraya koenigii, Alternanthera sessilis and many plants leaves extract have been studied [152]. Recently, P. nigrum leaves were stated to contain an important bioactive compound, which is involved in the nanoparticle synthesis by eco-friendly method. It shows a significant tool for antimicrobial agents in present and in a near future.
Flowers as source
[153] studied an eco-friendly method synthesis of gold nanoparticles by using rose petals. The extract medium contains abundant sugars and proteins. These functional compounds are the main sources for reduction of tetrachloroaurate salt into bulk GNPs. Likewise, Catharanthus roseus and Clitoria ternatea diverse groups of flowers are used for the metallic nanoparticle synthesis with desired sizes and shapes. The plant-synthesized nanoparticles have been effectively controlling harmful pathogenic bacteria and similarly the medicinal usable Nyctanthes arbortristis flowers of gold nanoparticles extract are synthesized via green chemistry method [154]. The aqueous extract of Mirabilis Jalapa flowers acts as a reducing agent and produced gold nanoparticles with ecofriendly method [155]
[153] studied an eco-friendly method synthesis of gold nanoparticles by using rose petals. The extract medium contains abundant sugars and proteins. These functional compounds are the main sources for reduction of tetrachloroaurate salt into bulk GNPs. Likewise, Catharanthus roseus and Clitoria ternatea diverse groups of flowers are used for the metallic nanoparticle synthesis with desired sizes and shapes. The plant-synthesized nanoparticles have been effectively controlling harmful pathogenic bacteria and similarly the medicinal usable Nyctanthes arbortristis flowers of gold nanoparticles extract are synthesized via green chemistry method [154]. The aqueous extract of Mirabilis Jalapa flowers acts as a reducing agent and produced gold nanoparticles with ecofriendly method [155]
Factors influencing the synthesis of metallic nanoparticles
During the course of biological synthesis of MNPs a number of controlling factors are involved in the nucleation and subsequent formation of stabilized NP. These factors include pH, reactants concentration, reaction time and temperature and details were given in Table 2 [156-160].
During the course of biological synthesis of MNPs a number of controlling factors are involved in the nucleation and subsequent formation of stabilized NP. These factors include pH, reactants concentration, reaction time and temperature and details were given in Table 2 [156-160].
Controlling factors | Influence on biological synthesis of MNPs | References |
pH | Variability in size and shape | [156] |
Reactants concentration | Variability in shape | [157] |
Reaction time | Increase in reaction time increases the size of MNPs | [158] |
Reaction temperature | Size, shape, yield and stability | [159-160] |
Table 2: Factors influencing the biological synthesis of MNPs [161].
The different hydrogen ion concentration is responding to the different size and shapes of nanoparticles formation [162] reported that Aloe vera extract produced Au–Ag core nanoparticles in various sizes and shapes by fluctuating the pH of the solvent medium. Similarly, biosynthesis of nanoparticles by alfalfa plant extract of the pH is retort for the size variation in the nanoparticles production. On the other hand, temperature is also one of the stimulating factors for the nanoparticles biosynthesis with different size and shapes. However, the study on AuNPs formation using leaf extract of Cymbopogon flexuosus [163] revealed that at high temperatures, it will lead to the formation of higher spherical NPs and nanotriangles, whereas lower reaction temperature mostly increases the nanotriangle formation. The difference in morphology is mainly percentage/amount of the salt solution in the reaction mixture. The concentration of salt could be altered with the reduction ability and sizes. Some environmental factors such as physical and chemical parameters controlled metallic crystal structure using the plant biomass as substrates [164]. In addition, the reduction reaction time (minutes–hours) is one of the factors to reduce the ions into bulk metal with variant shapes. The optimum time period produces high absorbance peak value to identify the higher concentration MNPs in the medium. It determined the employment of growth conditions and obvious formation of different size of MNPs such as spherical, triangular, hexagonal and rectangular [165].
Capping agents and their types
Capping agents play a very pivotal and versatile role in the NP synthesis. NPs can be functionalized and stabilized using capping agents to impart useful properties by controlling morphology, size and protecting the surface thereby preventing aggregation. Many surfactants have been reported to be used as capping agents for altering the desired shape and size of the MNPs but these are difficult to remove and do not easily degrade. Thus, the commercial surfactants are hazardous to the environment [166,167]. In the view of the limitation possessed by these chemicals, there is an urgent need to use environment-friendly capping agents and design green biochemical routes at laboratory and industrial level for the NP synthesis. There are different types of molecules that could act or be used as capping agents but some of the broadly classified green capping agents have been discussed below with their potential role.
Capping agents play a very pivotal and versatile role in the NP synthesis. NPs can be functionalized and stabilized using capping agents to impart useful properties by controlling morphology, size and protecting the surface thereby preventing aggregation. Many surfactants have been reported to be used as capping agents for altering the desired shape and size of the MNPs but these are difficult to remove and do not easily degrade. Thus, the commercial surfactants are hazardous to the environment [166,167]. In the view of the limitation possessed by these chemicals, there is an urgent need to use environment-friendly capping agents and design green biochemical routes at laboratory and industrial level for the NP synthesis. There are different types of molecules that could act or be used as capping agents but some of the broadly classified green capping agents have been discussed below with their potential role.
Biomolecules
The preparation of homogenous MNPs using biomolecules has recently gained interest due to their non-toxic nature and not involving harsh synthetic procedures. Amino acids act as an efficient reducing as well as capping agents to synthesize MNPs with unique structure. Maruyama and coworkers synthesized Au NPs with the size range of 4–7 nm using amino acids as capping agents. Among 20 different amino acids, they adopted L-histidine which was found to reduce tetraauric acid (AuCl4–) to Au NPs. The concentration of L-histidine was found to affect the size of MNPs; higher the concentration smaller the size of MNP. Moreover, the amino and carboxy groups present in the amino acids caused the reduction of AuCl4– and coating of MNP surface [168].
The preparation of homogenous MNPs using biomolecules has recently gained interest due to their non-toxic nature and not involving harsh synthetic procedures. Amino acids act as an efficient reducing as well as capping agents to synthesize MNPs with unique structure. Maruyama and coworkers synthesized Au NPs with the size range of 4–7 nm using amino acids as capping agents. Among 20 different amino acids, they adopted L-histidine which was found to reduce tetraauric acid (AuCl4–) to Au NPs. The concentration of L-histidine was found to affect the size of MNPs; higher the concentration smaller the size of MNP. Moreover, the amino and carboxy groups present in the amino acids caused the reduction of AuCl4– and coating of MNP surface [168].
In another interesting study, Au nanochains were prepared via facile single step within 15 min in the presence of glutamic acid and histidine amino acids [169]. The oriented attachment mechanism has been proposed where there is spontaneous self-organization of the adjacent particles at a planar interface as they share a common crystallographic orientation (Figure 13). The binding affinity of amino acids is found to be different for different facets of Au crystal. The fusion of MNPs along (111) facet revealed that binding affinity of amino acids along this facet might be weaker as compared to the other facets. The removal of amino acid molecules from (111) facet allows the linear aggregation of particles due to dipole–dipole interactions which arise as a result of the zwitterionic nature of amino acids [170].
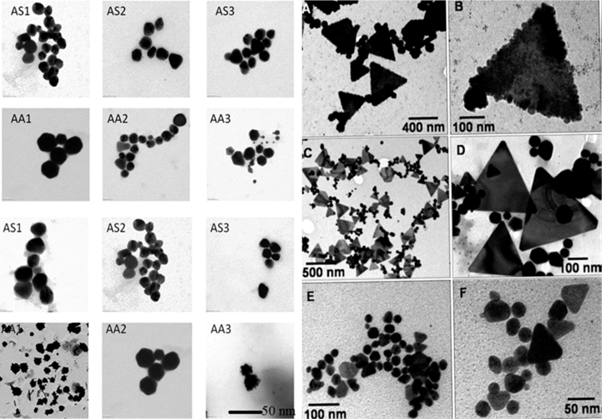
Figure 13: Illustration of Au nanochain and nanowire formation through dipole–dipole interaction due to the zwitterionic nature ofamino acids. Reproduced with permission [169].
Polysaccharides
Polysaccharides are a class of polymeric carbohydrate molecules with repeating units of mono or disaccharides linked together by glycosidic linkages. They act as capping agents in the MNP synthesis as they are low cost, hydrophilic, stable, safe, biodegradable and non-toxic. The synthesis is carried out in the presence of water as a solvent thus, eliminating the use of toxic solvents [171,172]. One of the distinguishing features of polysaccharides is that they sharply accelerate the kinetics of sol–gel processes due to their catalytic effect [173]. They not only have been found to modify the structure and morphology of TiO2 but have induced a different phase where rutile phase has been obtained in the presence of chitosan whereas anatase in the presence of starch [174].
Polysaccharides are a class of polymeric carbohydrate molecules with repeating units of mono or disaccharides linked together by glycosidic linkages. They act as capping agents in the MNP synthesis as they are low cost, hydrophilic, stable, safe, biodegradable and non-toxic. The synthesis is carried out in the presence of water as a solvent thus, eliminating the use of toxic solvents [171,172]. One of the distinguishing features of polysaccharides is that they sharply accelerate the kinetics of sol–gel processes due to their catalytic effect [173]. They not only have been found to modify the structure and morphology of TiO2 but have induced a different phase where rutile phase has been obtained in the presence of chitosan whereas anatase in the presence of starch [174].
Dextran is a complex branched polysaccharide composed of many glucose molecules with chains of varying lengths. It is hydrophilic, biocompatible, non-toxic and used for coating of many metal MNPs [175]. Spherical Au NPs of size _15 nm were synthesized in water using natural honey which acted as reducing as well as protecting agent. Fructose present in the honey was supposed to act as a reducing agent whereas proteins were responsible for the stabilization of the MNPs [176]. Cheng and coworkers synthesized Ag NPs within the size range of 2–14 nm using amino cellulose as reducing and capping agent. Aminocellulose is generally referred as aminodoxy derivative bearing nitrogen functional group attached directly to the cellulose backbone. It was predicted that at high temperature, reduction of Ag+ ions to Ag (0) was brought by cellulose [177]. Thus, polysaccharides have come up as one of the renewable green alternatives for the fabrication of NPs replacing toxic chemicals thereby saving the environment from their hazardous effects.
Feng., et al. explored the adsorption mechanism of amino acids and surfactants on to the (111) surface of gold using molecular dynamic simulation with the application of intermolecular potential CHARMM-METAL [178]. The molecules adsorbed onto the surface with energy between -3 and -26 kcal mol-1 and it correlated with the preferential degree of coordination of polarizable atoms (O, N, and C) to multiple epitaxial sites. The amino acids containing planar side groups such as Arg, Trp, Gln, Met, Tyr, Asn, and PPh3 with sp2 hybridization adsorbed strongly indicating a correlation with molecular size and geometry. The movement of the strongly bonded amino acids has been attributed to hopping mechanism where surface attached guanidinium group of Arginine moved from one favorable coordination site to another on the metal surface in the order of picoseconds (Figure 14). This adsorption mechanism could give the knowledge to control the assembly and growth of MNPs.
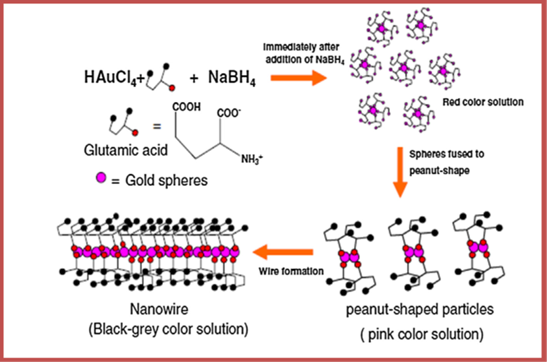
Figure 14: Representative snapshot of Arg on the Au 111 surface. The guanidinium group is found most of the time in a favorable coordination pattern with numerous epitaxial sites which leads to strong adsorption (pink highlights). Diffusion on the surface occurs by stepwise hopping of the guanidinium group to similar epitaxial environments. In the depicted conformation, Ca and Cg point upwards from the metal surface. Most water molecules are not shown for ease of visualization and the chloride counterion is located outside the visible range near the metal surface. Metal atoms are shown in decreasing diameter from the top atomic layer toward adjacent atomic layers. Reproduced with permission [178].
Metallic Nanoparticle Synthesis Using Microorganisms
Microorganisms have been shown to be important nanofactories that hold immense potential as ecofriendly and cost-effective tools, avoiding toxic, harsh chemicals and the high-energy demand required for physiochemical synthesis. Microorganisms have the ability to accumulate and detoxify heavy metals due to various reductase enzymes, which are able to reduce metal salts to metal nanoparticles with a narrow size distribution and, therefore, less polydispersity. Over the past few years, microorganisms, including bacteria (such as actinomycetes), fungi, algae and yeasts, have been studied extra- and intracellularly for the synthesis of metal nanoparticles. An array of biological protocols for nanoparticle synthesis has been reported using bacterial biomass, supernatant, and derived components.
Microorganisms have been shown to be important nanofactories that hold immense potential as ecofriendly and cost-effective tools, avoiding toxic, harsh chemicals and the high-energy demand required for physiochemical synthesis. Microorganisms have the ability to accumulate and detoxify heavy metals due to various reductase enzymes, which are able to reduce metal salts to metal nanoparticles with a narrow size distribution and, therefore, less polydispersity. Over the past few years, microorganisms, including bacteria (such as actinomycetes), fungi, algae and yeasts, have been studied extra- and intracellularly for the synthesis of metal nanoparticles. An array of biological protocols for nanoparticle synthesis has been reported using bacterial biomass, supernatant, and derived components.
Among the various methodologies, extracellular synthesis has received much attention because it eliminates the downstream processing steps required for the recovery of nanoparticles in intracellular methodologies, including sonication to break down the cell wall, several centrifugation and washing steps required for nanoparticle purification, and others. Moreover, metal-resistant genes, proteins, peptides, enzymes, reducing cofactors, and organic materials have significant roles by acting as reducing agents. Furthermore, these help in providing natural capping to synthesize nanoparticles, thereby preventing the aggregation of nanoparticles and helping them to remain stable for a long time, thus providing additional stability.
In recent research, bacteria, including Pseudomonas deceptionensis [179], Weissella oryzae [180], Bacillus methylotrophicus [181], Brevibacterium frigoritolerans [182], and Bhargavaea indica [183,184], have been explored for silver and gold nanoparticle synthesis. Similar potential for producing nanoparticles has been showed by using several Bacillus and other species, including Bacillus licheniformis, Bacillus amyloliquefaciens, Rhodobacter sphaeroides [185-187], Listeria monocytogenes, Bacillus subtilis, and Streptomyces anulatus [187,188]. Various genera of micro-organisms have been reported for metal nanoparticle synthesis, including Bacillus, Pseudomo-nas, Klebsiella, Escherichia, Enterobacter, Aeromonas, Corynebacterium, Lactobacillus, Pseudomonas, Weissella, Rhodobacter, Rhodococcus, Brevibacterium, Streptomyces, Tricho-derma, Desulfovibrio, Sargassum, Shewanella, Plectonemaboryanum, Rhodopseudomonas, Pyrobaculum, and others [135].
These investigations suggest that the main mechanism of the synthesis of nanoparticles using bacteria depends on enzymes [189]; for instance, the nitrate reductase enzyme was found to be responsible for silver nanoparticle synthesis in B. licheniformis. Rather than using bacteria, mycosynthesis is a straightforward approach for achieving stable and easy biological nanoparticle synthesis. Algae and most fungi containing important metabolites with higher bioaccumulation ability and simple downstream processing are easy to culture for the efficient, low-cost, production of nanoparticles [190]. Moreover, compared with bacteria, fungi have higher tolerances to, and uptake competences for, metals, particularly in terms of the high wall-binding capability of metal salts with fungal biomass for the high-yield production of nanoparticles [190,191].
Three possible mechanisms have been proposed to explain the mycosynthesis of metal nanoparticles: nitrate reductase action; electron shuttle quinones; or both [190]. Fungal enzymes, such as the reductase enzymes from Penicillium species and Fusarium oxysporum, nitrate reductase, and/-NADPH-dependent reductases, were found to have a significant role in nanoparticle synthesis [192], similarly to the mechanism found in bacteria. The synthesis of nanoparticles using actinomycetes has not been well explored, even though actinomycetes-mediated nanoparticles have good monodispersity and stability and significant biocidal activities against various pathogens [193].With the availability of sophisticated biochemical techniques and instruments, it is easy to identify the role and interaction of a particular biomolecule such as polysaccharides, proteins, enzymes present in the organism with NPs thereby comprehending the mechanism. The simple mechanism in Figure 15 explains that enzymes and functional groups present in the cell walls of algae form complexing agents with the precursors thereby, causing reduction and deposition of metal/metal oxide NPs at ambient conditions [194,195]. The different sources of waste materials for the green synthesis of MNPs was schematically represented in Figure 16.
The synthesis of silver, copper, and zinc nanoparticles using Streptomyces sp. has demonstrated that the reductase enzyme from Streptomyces sp. has a vital role in the reduction of metal salts [196]. Similar to other microorganisms, yeasts have also been widely investigated for the extracellular synthesis of the nanoparticles on a large scale, with straightforward downstream processing [197-200]. Further-more, virus-mediated synthesis of nanoparticles is also possible. However, most microorganism-based syntheses for nanoparticles are slow with low productivity, and the recovery of nanoparticles requires downstream processing. Furthermore, problems related to microorganism-based synthesis for nanoparticles also include the complex steps, such as microbial sampling, isolation, culturing, and maintenance.
Preparation of Metallic MNPs
The controlled growth of NPs in solution is believed to be kinetically controlled process where low energy faces of any crystal results into a particular shape. The energy and growth rate of a crystal can be controlled by the introduction of a suitable templating agent or a surfactant, which lowers the interfacialenergy [201,202]. Till now, different commercial surfactants have been used as templates and capping agents for the synthesis of MNPs with varied morphologies. However, the problem is the removal and complete biodegradation of these chemicals. Nowadays, more and more research is converged on to the green synthesis employing environment-friendly and bioinspired approaches. By knowing the capability of naturally occurring biomolecules to modify the shape or size of a crystal, MNPs of superior quality can be manufactured. The use of different species of algae in the synthesis of metallic MNPs has stimulated the researchers to come up with ‘nature-friendly’ methodologies.
The controlled growth of NPs in solution is believed to be kinetically controlled process where low energy faces of any crystal results into a particular shape. The energy and growth rate of a crystal can be controlled by the introduction of a suitable templating agent or a surfactant, which lowers the interfacialenergy [201,202]. Till now, different commercial surfactants have been used as templates and capping agents for the synthesis of MNPs with varied morphologies. However, the problem is the removal and complete biodegradation of these chemicals. Nowadays, more and more research is converged on to the green synthesis employing environment-friendly and bioinspired approaches. By knowing the capability of naturally occurring biomolecules to modify the shape or size of a crystal, MNPs of superior quality can be manufactured. The use of different species of algae in the synthesis of metallic MNPs has stimulated the researchers to come up with ‘nature-friendly’ methodologies.
Degradation of hazardous organic chemicals and dyes is a crucial problem. Many techniques such as activated carbon sorption, electrocoagulation, UV degradation and redox treatments are used but these are not found to be very efficient and cost effective. Therefore, there is an urgent need of improved ways to degrade chemicals as well as treatment of wastewater.
The extracellular synthesis of monodispersed Au NPs has been achieved in a short duration from marine alga Sargassum wightii Greville. The alga caused the reduction of auric chloride solution and the AuNPs were stable in solution which isimportant from biological prospective [203]. Stoechospermum marginatum is brown algae with a high metal binding capacity and constitutes proteins, vitamins, amino acids, fatty acids, minerals and trace elements. Au NPs were prepared from this algal biomass with a reaction time of 10 min [204]. AuNPs have been reported to be intracellularly synthesized in the suspensions encapsulated within silica gels in the presence of Klebsormidium flaccidium algal cells giving rise to ‘‘living” bio-hybrid material. TEM images show algal cells before and after the addition of gold (Figure 17).
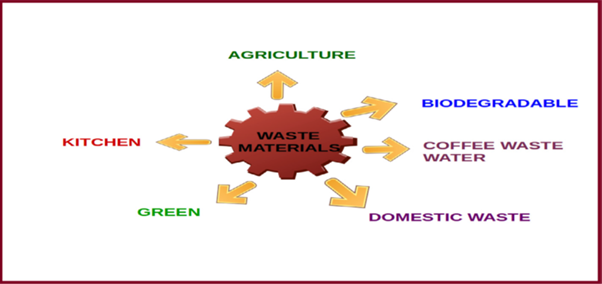
Figure 17: TEM images of Kf cells within silica before (a) and after (b) gold addition. Gold colloids could be found within the cells in the thylakoids (c), in the cell EPS (d) and in the surrounding silica gel (e) ED pattern of dark dots with examples of attribution to the cfc Au structure (f). Reproduced with permission [205].
The cells are surrounded by the colloidal silica which is not in direct contact with the cells thereby maintaining the ability to synthesize Au NPs intracellularly. The proximity of silica cells with cell membranewas found to be dependent on the physiological state of algae. This pathway proves to be one of the promising ’green’ routes for the synthesis of MNPs [205]. The diversity in the algae species has led to their exploitation and even the edible forms of algae are used in the synthesis of metallic NPs.
Au NPs have paved a way in the field of science and technology due to their enormous vital applications as optical, electronic, catalytic, biosensors and drug carriers. They exhibit excellent antimicrobial and antioxidant properties as their surface is functionalized [206-211]. The chemical methods employ solvents, which are toxic in nature and pose a limitation for the use of NPs in the medical field. The biomatrix of freshwater, edible red alga Lemanea fluviatilis has been used to accomplish the synthesis of Au NPs. The proteins present in the alga acted as templating as well as stabilizing agent, thereby avoiding the use of surfactants which are difficult to remove [212]. Prasiola crispa is another freshwater green algae which is used for the one step biosynthesis of Au NPs in the size range of 5–25 nm via reduction of chloroauric acid [213]. It has been reported that algal morphology along with high pH, metal ion concentration and biomass concentration are important factors for the monodispersity of the MNPs. However, with time, on exposure of MNP the toxicity levels against alga increase, but the synthesized NPs show no toxicity when tested with cell lines of normal human cell [214]. Thus, algal-MNPs interactions are very important considering the effective use of MNPs in drug delivery (Figure 18).
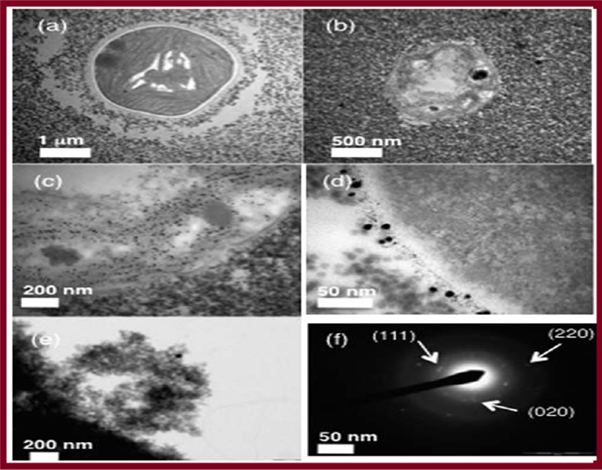
Figure 18: Microphotographs of Au3+ exposed algal filaments showing changes in morphological and reproductive behavior at different time points. (a) Akinete formation within 12h; (b) series of akinetes at 24h; (c) Au NP deposition started in vegetative cells and akinetes after 24h; (d) cell wall thickening after 48h; (e) giant cell with complete Au NP deposition after 48h; (f) pyknotic cell after 48h; (g) Au NP deposition at the periphery of cells; (h) degradation of chlorophyll and Au NP deposition within cell after 48h; (i) purple colored filament after 72h. Scale bars, 20 lm (inset showing control filaments; scale bar, 40 lm). Reproduced with permission [214].
Recently, several studies have reported natural polymers such as chitosan, starch and tannic acid as reducing agents for the synthesis of silver and gold nanoparticles [215, 216]. A vast array of biological resources including plants, algae, fungi, yeast, bacteria, and viruses has been studied so far for the intra and extracellular synthesis of silver, gold, platinum and titanium nanoparticles in different sizes and shapes were tabulated in Table 3. The major drawback of metal nanoparticles synthesis using plant extracts as reducing and stabilizing agent. This differs due to significant variation of biochemical compositions present in the plant extract of the same species differ from other part of the world. Therefore, identifying the biomolecules responsible for mediating the nanoparticles synthesis is a problem to overcome [217].
Sources | Type of nanoparticles | Location | Size (nm) |
A. Bacteria | |||
Pseudomonas aeruginosa | Au | Extracellular | 15~30 |
Pseudomonas stutzeri | Ag | Intracellular | 200 |
Bacillus subtilis | Ag &Au | Intra & Extracellular | 5~10 |
Shewanella oneidensis | U | Extracellular | 150 |
Lactobacillus sp. | Ag & Au | Intracellular | 60 |
Escherichia coli | CdS | Intracellular | 2~5 |
Clostridium thermoaceticum | CdS | Intra & Extracellular | 2~5 |
Rhodopseudomonas capsulate | Au | Extracellular | 10~20 |
Escherichia coli DH5α | Au | Intracellular | 25~33 |
Thermomonospora spp. | Au | Extracellular | 8 |
Streptomyces albidoflavus | Ag | Intracellular | 10~14 |
Klebsiella pneumonia | Ag | Extracellular | 5~32 |
B. Virus | |||
Tobacco mosaic virus (TMV) | SiO2, CdS, PbS, Fe2O3 | Intra & Extracellular | 45~80 |
M13 bacteriophage | ZnS and CdS | Intra & Extracellular | 50~100 |
C. Fungi | |||
Phoma sp. 3.2883 | Ag | Extracellular | 71~74 |
Fusarium oxysporum | Ag | Extracellular | 5~15 |
Verticillium | Ag | Intracellular | 25 |
Aspergillus fumigates | Ag | Extracellular | 5~25 |
Trichoderma asperellum | Ag | Extracellular | 13~18 |
Phaenerochaete chrysosporium | Ag | Extracellular | 50~200 |
Fusarium oxysporum | Magnetite | Extracellular | 20~50 |
D. Yeast | |||
Pichia jadinii | Au | Intracellular | 100 |
Torulopsis | CdS | Intracellular | 2~5 |
Candida glabrata | CdS | Intracellular | 200 |
Schizosaccharomyces pombe | CdS | Intracellular | 1~1.5 |
E. Algae | |||
Scencedesmus sp. | Ag | Extracellular | 15~20 |
Chlorella vulgaris | Au | Extracellular | 9~20 |
F. Plant | |||
Alfalfa sprouts | Ag | Intracellular | 2~20 |
Cinnamomum camphora | Ag and Au | Extracellular | 55~80 |
Azadirachta indica (Neem) | Ag/Au | Extracellular | 50~100 |
Geranium leaves plant extract | Ag | Extracellular | 16~40 |
Avena sativa (Oat) | Au | Extracellular | 5~85 |
Aloe vera | Au | Extracellular | 50~350 |
G. Human cell lines | |||
SiHa | Au | Intracellular | 20~100 |
HeLa | Au | Intracellular | 20~100 |
SKNSH | Au | Intracellular | 20~100 |
HEK-293 | Au | Intracellular | 20~100 |
Table 3: Biological synthesis of metal nanoparticles using various organisms [218].
Preparation of Metal oxide NPs
Metal oxides are widely explored and studied class of inorganic solids due to a wide variety of structures, properties and exceptional phenomenon exhibited by their NPs. Transition metal oxides have been used in numerous industrial applications. NPs, nano-powders and nanotubes play a significant role in industry, environmental remediation, and medicine and even in household applications. One dimensional (1D) metal oxide nanostructures are ideal systems for exploring size and morphology dependent applications and have become the focus of current research efforts in the field of nanoscience and nanotechnology. Metal oxides are commonly available and present in different forms possessing special shapes, composition, structures, chemical and physical properties [219].
Metal oxides are widely explored and studied class of inorganic solids due to a wide variety of structures, properties and exceptional phenomenon exhibited by their NPs. Transition metal oxides have been used in numerous industrial applications. NPs, nano-powders and nanotubes play a significant role in industry, environmental remediation, and medicine and even in household applications. One dimensional (1D) metal oxide nanostructures are ideal systems for exploring size and morphology dependent applications and have become the focus of current research efforts in the field of nanoscience and nanotechnology. Metal oxides are commonly available and present in different forms possessing special shapes, composition, structures, chemical and physical properties [219].
Different synthetic methods such as hydrothermal, solvothermal, microwave, vapor deposition, seed mediated, spray pyrolysis, wet-chemical have been employed for the preparation of metal oxide NPs with diverse morphology and size [220-223]. The reports on the biosynthesis of metal oxide NPs are very few. The research is now being shifted from conventional synthetic methods to biosynthesis process where microorganism could be employed for synthesizing NPs. Very few articles have reported the biosynthesis approaches, especially using algae. Copper oxide NPs have been prepared from brown alga, Bifurcaria bifurcata extract with a dimension between 5 and 45 nm (Figure 19). The NPs were stable in solution, which is promising for their use in biomedical applications. These NPs exhibited antibacterial activity against bacterial strains of Enterobacter aerogenes and Staphylococcus aureus [224].
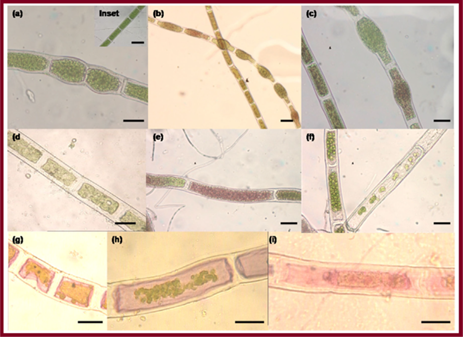
Figure 19: TEM image of CuO NPs synthesized from the alga extract. Reproduced with permission [224].
The extracts of green seaweed Caulerpa peltata, red Hypnea Valencia and brown Sargassum myriocystum were also used for the biosynthesis of ZnO NPs. The NPs with different morphologies such as spherical, triangular, radial, hexagonal, rod and rectangular were formed with size range of 76–186 nm. The water soluble pigments present in the extract were found to be responsible for the reduction and stabilization of the NPs [225]. AFM study of ZnO NPs revealed that change in temperature parameter remarkably affected the size and morphology of the particles (Figure 20).
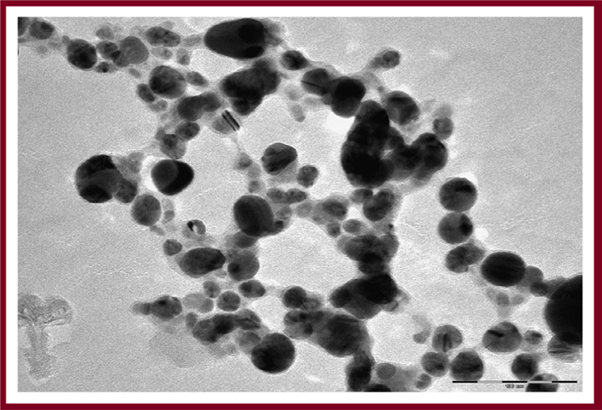
Figure 20: AFM results of ZnO NPs 2D and 3D images. (a) Unfiltered AFM image showing topographical 2D image of ZnO NPs (b) 3D image of synthesized (c) filtered 2D AFM image of ZnO nanoparticles (d) particle size distribution of ZnO NPs. Reproduced with permission [225].
The polysaccharides present in the aqueous extract of Sargassum muticum caused the reduction of ferric chloride solution, thereby leading to the formation of ferric oxide (Fe3O4) NPs with cubic morphology and average diameter of 18 ± 4 nm [226]. S. muticum is algal seaweed which is also used as functional food consisting of large quantities of lipids, minerals, vitamins and many other bioactive substances such as polysaccharides, proteins and polyphenols. These biomolecules are effective against cancer, diabetes, thrombosis, obesity, and other degenerative diseases [227-230] and act as reducing as well as capping agents. ZnO nanomaterials are considered biocompatible for the medical applications.
Therefore, research is carried out to develop algae mediated one-step, green and eco-friendly approach. Recent report on the synthesis via aqueous extract of brown alga S. muticum has indicated the formation of pure ZnO NPs in the size range of 30–57 nm with hexagonal crystal structure [231] (Azizi., et al. 2014). Francavilla et al., designed a feasible protocol for the synthesis of ZnO NPs using Gracilaria gracilis, an edible form of algae [232]. This protocol involved the solid state grinding of zinc precursors including a biomass extracted from microalga followed by thermal decomposition in the absence of any solvent. The synthesized NPs were found to degrade phenol efficiently (Figure 21A). ZnOAG were agar synthesized and ZnOAA alginic acid as compared to commercial P25 Evonik. The photocatalytic activity was enhanced in the case of agar synthesized (52% phenol degradation, Figure 21B) whereas the degradation of phenol was less than 35% in the case of ZnOAA. The contribution to enhanced photocatalytic activity in this case is still under study but the methodology could be applied widely for the synthesis of other metal oxide NPs.
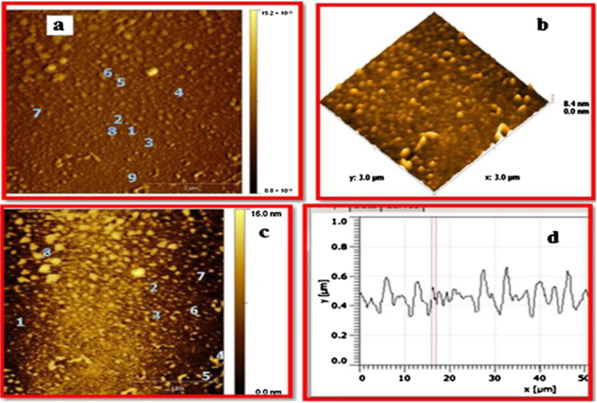
Figure 21: (A) Phenol degradation efficiency (measured as the relative concentration of phenol (C/Co) over time) of ZnOAA 1: 16 alginic acid, ZnOAG 1: 2 ex. agar and P25 Evonik, (B) Photocatalytic degradation curves of phenol for ZnOAA 1: 16 alginic acid, ZnOAG 1: 2 ex. agar and P25 Evonik. Reproduced with permission [232].
The mechanism for the formation of MNPs in the presence of algae is yet to be fully understood. The algal membranes consist of biomolecules such as polysaccharides, proteins and enzymes, which catalyze the reduction of metal salt precursors into metal or metal oxide NPs. Since, these are large molecules and amphiphilic in nature, they act as surfactant molecules which causes not only concentration buildup of the surfactant at the surface and reduction of the surface tension, but also the orientation of the molecule at the surface [233,234]. They act as capping agents and thus, reduce the interfacial energy. The interaction of these biomolecules with the NPs is also an important aspect under consideration.
Commercial applications of biosynthesized nanoparticles
Nanoparticles (NPs) are particles that range in size between 1 and 100 nanometres in diameter. This dimensional feature gives specific properties or behaviours to these materials. Because of the many innovative applications suggested by these physical, chemical or biological properties, nanomaterials represent a field of scientific and technical research in full expansion. Indeed, the functional properties of the MNPs mainly depend on their physicochemical characteristics: these particles may exist in aggregated or discrete form and can be hexagonal, spherical, tubelike, or irregularly shaped. Thus, their potential toxicity in the human body will particularly depend on their physicochemical properties (size, shape, crystal structure or not, surface charge, solubility, etc.) [78].
Nanoparticles (NPs) are particles that range in size between 1 and 100 nanometres in diameter. This dimensional feature gives specific properties or behaviours to these materials. Because of the many innovative applications suggested by these physical, chemical or biological properties, nanomaterials represent a field of scientific and technical research in full expansion. Indeed, the functional properties of the MNPs mainly depend on their physicochemical characteristics: these particles may exist in aggregated or discrete form and can be hexagonal, spherical, tubelike, or irregularly shaped. Thus, their potential toxicity in the human body will particularly depend on their physicochemical properties (size, shape, crystal structure or not, surface charge, solubility, etc.) [78].
Classical approaches of metals
Anciently, the gold metal is known as a symbol of power and wealth. The gold metal is used in different forms to improve the human health ever since. Even today, the biological aspects of metallic gold nanoparticles (GNPs) are very useful to human health and cosmetics applications [235]. In the 18th century, Egyptians used gold metal solubilized water for mental and spiritual purification. The restorative property of gold is still honoured in rural villages, where peasants cook their rice with a gold pellet to replace the minerals in the body via food intake. Traditionally, silver metal is used to control bodily infection and prevent food spoilage. Silver is used as wound healer agents and ulcer treatment [236]. In fact, nowadays the colloidal silver nanoparticles have been used as antimicrobial agent, wound dressing material, bone and tooth cement and water purifier as well [237].
Anciently, the gold metal is known as a symbol of power and wealth. The gold metal is used in different forms to improve the human health ever since. Even today, the biological aspects of metallic gold nanoparticles (GNPs) are very useful to human health and cosmetics applications [235]. In the 18th century, Egyptians used gold metal solubilized water for mental and spiritual purification. The restorative property of gold is still honoured in rural villages, where peasants cook their rice with a gold pellet to replace the minerals in the body via food intake. Traditionally, silver metal is used to control bodily infection and prevent food spoilage. Silver is used as wound healer agents and ulcer treatment [236]. In fact, nowadays the colloidal silver nanoparticles have been used as antimicrobial agent, wound dressing material, bone and tooth cement and water purifier as well [237].
The applications of these materials are numerous and now remarkably significant in human life and in industries [238]. Thus, MNPs are now included in many industrial processes and are involved in the composition of a wide variety of products and systems used in everyday life (food and drinks, sunscreens, textiles, etc.). Nanomaterials appear in different areas such as health and fitness, electronics and computers, packaging, motorcar, building, chemicals, energy and environment. This rapid expansion of such applications causes an increase in the population exposure to manufactured nanomaterials, i.e. produced intentionally. Their absorption, distribution, metabolism and excretion appear to be different compared to a conventional chemical entity, due to their specific characteristics.
Today, the wide distribution of MNPs through a multitude of consumer products raises questions about exposure of consumers and the general population to manufactured nanomaterials. Favourable modification of the properties of materials by changing their size is also possible, enhancing their profitability. Today, nanotechnology is used in a broad spectrum of scientific fields (biotechnology, medicine, pharmacy, ecology, electronics and others). The possibility of applying nanomaterials for use in agriculture, veterinary medicine, the food industry and cosmetology is also being explored [239]. The group of materials of particular interest includes: nanoparticles of silver, gold, zinc, selenium, titanium dioxide and carbon nanotubes [240]. In addition, it is expected that in the near future, the scope of research will enable materials to be obtained with previously unknown properties. Medical bioengineering is an area where nanotechnology has found many applications [241]. In this area, nanotechnology is mainly used in the field of diagnostic tests – as a tool for the detection of diseases and their imaging and monitoring of pharmacological therapy (mainly the system of drug delivery and disposition in the human body) [242]. Nanomaterials are increasingly an integral part of orthopaedic implants and scaffolds for tissue bioengineering. The main advantage of such solutions is the ability to manipulate the properties of biomaterial surfaces on a nanometric scale. That results in the unmistakable increase in the level of biocompatibility of implants used [243]. The beauty industry is another sector in which nanotechnology is used [244,245]. In this field, it is very important to protect the products against microbial contamination which may occur during production of cosmetics or their storage.
Before nanotechnology permanently penetrated the cosmetic industry, organic compounds such as parabens [246] and phenoxyethanol [247] had been used to control unwanted microbial flora. Studies revealed the irritant effects of these types of preservatives, especially parabens, in relation to epidermis [248]. What is more, their increased susceptibility to UV light has been confirmed [249,250]. The harmful preservatives have been partially replaced by metal nanoparticles, in particular silver nanoparticles [251]. Nanotechnology is also used in information technology. In recent decades a term “computers of new generations” has become a common phenomenon. These are the computers which are produced with the use of nanomaterials as component elements that increase efficiency and energy savings of these devices. In 2007 IBM’s research team published the results of using self-assembling nanostructures in the production of chips.
It was found that such a novel solution allows an increase the current flow of up to 35% in the chips. The company estimates that a similar application of nanomaterials will quadruple the number of chip transistors, so that future computers will work more efficiently [252]. The properties of nanomaterials also allow using them in the energy sector. The application of a new generation of catalysts, whose construction is based on nanostructures, results in a significant reduction in energy consumption. Studies carried out during the production of biomass fuels revealed that the use of nanoporous membranes facilitates an increased efficiency of the process. It was noted that no other ways of reducing processing costs and increasing the yield of energy processes are as efficient as using nanostructured materials [252].
Nanoparticles have also found an application in the industry of paints and coatings. For example, paints and coatings containing nanomaterials are used in the aerospace, automotive, construction and hygiene industries. By introducing nanoparticles in the structure of materials, it is possible to give products antibacterial and anticorrosion properties and protection against UV radiation. The resultant structures can also be easier to clean. Sigma Aldrich is currently the leading supplier of nanomaterials. BASF and Evonik Degussa are its close competitors, in particular in the offer of nanomaterials in cosmetics and personal hygiene (Figure 22 and Table1) [53].
Catalytic applications and waste water treatment
Recently, nanoproducts have immense applications in day to day life. There are also various eco-friendly nanoproducts available in commercial market with high efficiency such as water purifier, bone and teeth cement, facial cream and homemade products [253]. For instance, silver, silica and platinum nanoparticles have various applications in personal care and cosmetics and they are used as ingredients in various products such as sunscreens, anti-ageing creams, toothpastes, mouthwash, hair care products and perfumes [254]. The silica nanomaterials are used as ingredients in various commercial products. Also, the modified silica nanomaterials are used as excellent pesticide control and it is used in a variety of non-agricultural applications.
Recently, nanoproducts have immense applications in day to day life. There are also various eco-friendly nanoproducts available in commercial market with high efficiency such as water purifier, bone and teeth cement, facial cream and homemade products [253]. For instance, silver, silica and platinum nanoparticles have various applications in personal care and cosmetics and they are used as ingredients in various products such as sunscreens, anti-ageing creams, toothpastes, mouthwash, hair care products and perfumes [254]. The silica nanomaterials are used as ingredients in various commercial products. Also, the modified silica nanomaterials are used as excellent pesticide control and it is used in a variety of non-agricultural applications.
Biosynthesized MNPs exhibit interesting size dependent catalytic properties due to high surface-to-area volume ratio. Pd NPs synthesized using soya leaf extract caused the degradation of azo dyes [255]. Fe3O4 NPs coated with soluble bio-based products (SBO) efficiently adsorbed crystal violet (CV) dye used as a model pollutant (Figure 23A). Thus, these MNPs could be used for the removal of pollutants in the water [256]. The effect of pH on the removal of CV dye with NPs was studied by carrying out sorption experiments. It was observed that as pH was increased the % removal of dye also increased (Figure 23B).
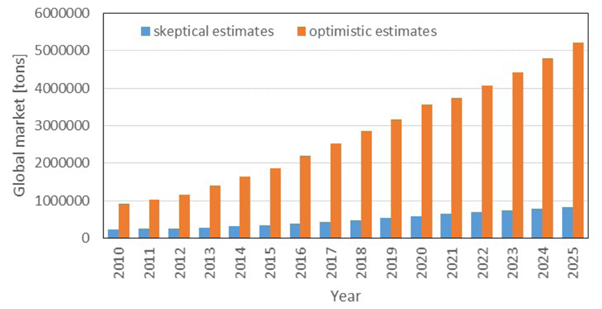
Figure 23: (A) Fe3O4 NPs coated with SBO (B) CV removal obtained with NP/0.5 at different PHS. [CV] 0 = 10 mg L-1; [NP/0.5]0 = 150 mg L-1. Reproduced with permission [256].
Spherical Pd NPs synthesized within green microalgae (Chlorella vulgaris) acted as a catalyst for Mizoroki–Heck cross-coupling reaction (Figure 24). They were synthesized via photosynthetic reaction and thus provide a green route of synthesis for other MNPs [257]. Pd NPs have also been reported to synthesize directly on alginic acid (AA) and seaweed (Laminaria digitata, a brown alga with the common name Oarweed). The reaction of iodobenzene and methyl acrylate was used to determine the catalytic activity. It was noted that 75% yield was obtained after 20 min in the presence of Pd NPs with a reusability of 2–3 times [258].
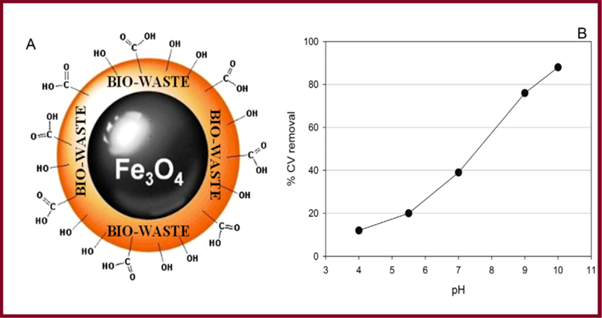
Figure 24: Palladium NP synthesis by photosynthetic green microalgae, and their uptake on an electrospun chitosan mat for use as a catalyst in Mizoroki–Heck reactions. The left stage shows a combination of mechanisms taking place within the photosynthetic organisms, resulting in the production of reducing agents. (ADP: adenosine diphosphate, ATP: adenosine triphosphate, FD: ferredoxin, NADP+: oxidized form of nicotinamide adenine dinucleotide phosphate, NADPH: reduced form of nicotinamide adenine dinucleotide phosphate, PGA: phosphoglycolic acid, RuBisCO: rubilose biphosphate carboxylase). NADPH is likely one of the main reducing agents for the reduction of Na2 [PdCl4], which is partially oxidized as a result of aerobic culture conditions. Reproduced with permission [257].
Biosensing applications
The biosensing applications of algae and waste mediated synthesized MNPs are under study and would be preferred over commercially synthesized MNPs. Here, in brief, biosensing ability of MNPs synthesized from other sources has been discussed which would make MNPs derived from algae and waste materials a better choice. Biosynthesized Au NPs have proved to be very important tool for hormone (HCG) detection in pregnant women urine sample [259]. Adrenaline acts as a drug which is widely used in the treatment of allergies, heart attack, asthma and cardiac surgery. Therefore, detection of adrenalin is becoming an active area of research from medical point of view. Gold nanoplasmonic [260] substrates with high sensitivity and spectral reproducibility are key components of molecular sensors based on surface-enhanced Raman scattering (SERS).
The biosensing applications of algae and waste mediated synthesized MNPs are under study and would be preferred over commercially synthesized MNPs. Here, in brief, biosensing ability of MNPs synthesized from other sources has been discussed which would make MNPs derived from algae and waste materials a better choice. Biosynthesized Au NPs have proved to be very important tool for hormone (HCG) detection in pregnant women urine sample [259]. Adrenaline acts as a drug which is widely used in the treatment of allergies, heart attack, asthma and cardiac surgery. Therefore, detection of adrenalin is becoming an active area of research from medical point of view. Gold nanoplasmonic [260] substrates with high sensitivity and spectral reproducibility are key components of molecular sensors based on surface-enhanced Raman scattering (SERS).
In this work, a confocal Raman microscope and several types of gold nanostructures (arrays of nanodiscs, nanocones and nanodisc dimers) prepared by hole-mask colloidal lithography (HCl) to determine the sources of variability in SERS measurements. The significant variations in the SERS signal can originate from the method of deposition of analyte molecules onto a SERS substrate. While the method based on incubation of SERS substrates in a solution containing the analyte yields a SERS signal with low variability, the droplet deposition method produces a SERS signal with rather high variability.
Variability of the SERS signal of a single nanoparticle [260] was determined from the statistical analysis of the SERS signal in short-range Raman maps recorded using different sized laser spots produced by means of different objectives. The number of nanoparticles located within the laser spot can be a source of substantial SERS signal variability, especially for high-magnification objectives. This indicates that SERS substrates prepared by HCl exhibit high SERS enhancement and excellent homogeneity (about 20% relative standard deviation from short-range maps). The nanocone arrays are shown (Figure 25) to provide the highest SERS enhancement, the lowest relative level of fluorescence background, and slightly better homogeneity when compared with arrays of nanodisc dimers or single nanodiscs.
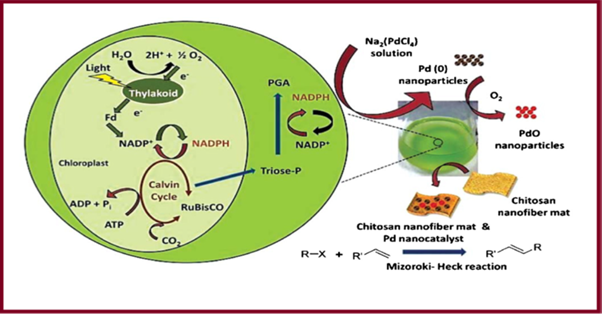
Figure 25: Gold nanostructures fabricated by hole-mask colloidal lithography as potential substrates for SERS sensors.
Copper oxide nanoparticles (nCOPs) having octahedral morphology, synthesized through hydrazine reduction reaction were employed to formulate an epoxy based novel nanocomposite. The synthesis of copper oxide nanoparticles [261] was carried out in polyethylene glycol medium to enhance their interfacial adhesion with the epoxy matrix. The extent of conservation of the crystalline nature and octahedral morphology of the nCOP in its epoxy nanocomposites was confirmed by X-ray diffraction and electron microscopy analysis.
The mechanical properties including tensile, impact, fracture toughness and surface hardness of epoxy–nCOP nanocomposites were evaluated as a function of nCOP content (Figure 26). The maximum enhancement in strength, modulus, impact strength, fracture toughness and surface hardness of epoxy–nCOP nanocomposites was observed for 5 phr nCOP content. This may be due to the strong interaction between the nCOP and epoxy chains at this composition arising from its fairly uniform dispersion. A quantitative measurement of constrained epoxy chains immobilized by the nCOP octahedra was carried out [261] using dynamic mechanical analysis.
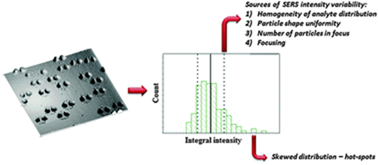
Figure 26: Copper oxide nanoparticles in an epoxy network: microstructure, chain confinement and mechanical behaviour.
The enhancement in the storage modulus is related to the amount of the added nCOP as well as the volume of the constrained epoxy chains in the proximity of nCOP. The behaviour of epoxy–nCOP nanocomposites in this study has been explained by proposing a mechanism based on the distribution of nCOP domains in the epoxy matrix and the existing volume of constrained epoxy chains. Pt NPs have been acted as a novel biosensor with high sensitivity for the determination of adrenaline [262]. Nanoscale Au–Ag alloy prepared via chloroplasts exhibited high electrocatalytic activity for 2-butanone at room temperature thereby providing a platform for the development of biosensor capable of detecting cancer at early stages [263]. Recently, attention has been focused on the synthesis and application of nanocomposites for supercapacitors [264], which can have superior electrochemical performance than single structured materials. Here, we report a carbon-coated TiO2/Co3O4 ternary hybrid nanocomposite (TiO2@C/Co) electrode for supercapacitors. A carbon layer was directly introduced onto the TiO2 surface via thermal vapor deposition (Figure 27). The carbon layer provides anchoring sites for the deposition of Co3O4, which was introduced onto the carbon-coated TiO2 surface by hydrazine and the thermal oxidation method.
The TiO2@C/Co electrode exhibits much higher charge storage capacity relative to pristine TiO2, carbon-coated TiO2, and pristine Co3O4, showing a specific capacitance of 392.4 F g−1 at a scan rate of 5 mV s−1 with 76.2% rate performance from 5 to 500 mV s−1 in 1 M KOH aqueous solution electrolyte. This outstanding electrochemical performance can be attributed to the high conductivity and high pseudo-capacitive contributions of the nanoscale particles. To evaluate the capacitive performance of a supercapacitor device employing the TiO2@C/Co electrode, we have successfully assembled TiO2@C/Co//activated carbon (AC) asymmetric supercapacitors. The optimized TiO2@C/Co//AC supercapacitor could be cycled reversibly in the voltage range from 0 to 1.5 V, and it exhibits a specific capacitance of 59.35 F g−1 at a scan rate of 5 mV s−1 with a specific capacitance loss of 15.4% after 5000
Charge–discharge cycles. These encouraging results show great potential in terms of developing high-capacitive energy storage devices for practical applications [264]. Three-dimensional (3D) interconnected carbon nanofibrous mats containing well-dispersed MoO2+δ nanocrystals are fabricated through a facile electrospinning route, (Figure 28) followed by thermal treatment in N2. The resulting nanostructured monolithic [265] hybrid mat made of C/MoO2+δ nanofibers exhibits superior Li-storage performances, when evaluated as a freestanding anode material. At a current density of 200 mA g−1, a reversible capacity as high as 876.9 mA h g−1 is achieved after 250 cycles.
A capacity of 447.9 mA h g−1 could still be maintained after 1000 cycles even at a high current density of 2000 mA g−1, indicating high rate capability and cyclability. The attractive electrochemical performances of the as-obtained 3D C/MoO2+δ networks may benefit from the synergistic effects of the unique nanoarchitectures and the integrity of the electrodes. Monodispersed MoO2+δ nanocrystals encapsulated in carbon nanofibers not only provide interfacial storage but also improve the transport kinetics of electrons and lithium ions [265].
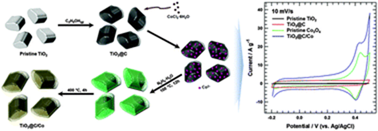
Figure 28: Direct planting of ultrafine MoO2+δ nanoparticles in carbon nanofibers by electrospinning.
Cosmetics
The metal nanoparticles are used as a preservative agent in food and cosmetic industries. New dimension of metallic nanoparticles is used for different commercial applications mainly cosmetics, pharma coating materials and food preservatives [251,266]. The nanosized metal nanoparticles such as gold, silver and platinum are broadly being applied for various commercial products such as shampoo, soap, detergent, and shoes. The chemical ingredients are mostly synthetic, and it causes side effects to human being. As a result, the green metallic nanoparticles are alternative for preservative agents in healthcare and food industries.
The metal nanoparticles are used as a preservative agent in food and cosmetic industries. New dimension of metallic nanoparticles is used for different commercial applications mainly cosmetics, pharma coating materials and food preservatives [251,266]. The nanosized metal nanoparticles such as gold, silver and platinum are broadly being applied for various commercial products such as shampoo, soap, detergent, and shoes. The chemical ingredients are mostly synthetic, and it causes side effects to human being. As a result, the green metallic nanoparticles are alternative for preservative agents in healthcare and food industries.
Nanoparticles in food industry
Silver metal is a highly heat conducting material because of that, nano-Ag is used in various mechanical devices. It is mainly used in heat liable instrumentation such as PCR lid and UV-spectrophotometer. The parts of instrumentation are made by nanosilver which is used as coated materials. It is highly stable in high temperature and without interference to the samples [267]. In food industries, the food products gets high microbial contamination due to their various open scale processes such as in manufacturing, processing and shipping of raw materials. Therefore, there is a need to develop a cost effective biosensor to evaluate the quality of the products. The metallic nanoparticles have been developed as biosensors and it effectively detects pathogen and monitors the different stages of contaminant with low cost.
Silver metal is a highly heat conducting material because of that, nano-Ag is used in various mechanical devices. It is mainly used in heat liable instrumentation such as PCR lid and UV-spectrophotometer. The parts of instrumentation are made by nanosilver which is used as coated materials. It is highly stable in high temperature and without interference to the samples [267]. In food industries, the food products gets high microbial contamination due to their various open scale processes such as in manufacturing, processing and shipping of raw materials. Therefore, there is a need to develop a cost effective biosensor to evaluate the quality of the products. The metallic nanoparticles have been developed as biosensors and it effectively detects pathogen and monitors the different stages of contaminant with low cost.
Pharmaceutical and biomedical applications of MNPs
The use of nanotechnology in medicine offers some exciting possibilities. Some techniques are only imagined, while others are at various stages of testing, or actually being used today. Nanotechnology in medicine involves applications of nanoparticles currently under development, as well as longer range research that involves the use of manufactured nano-robots to make repairs at the cellular level (sometimes referred to as nanomedicine). Whatever you call it, the use of nanotechnology in the field of medicine could revolutionize the way we detect and treat damage to the human body and disease in the future, and many techniques only imagined a few years ago are making remarkable progress towards becoming realities. Being super paramagnetic in nature, iron and iron oxide NPs find extensive usage in biomedical applications such as cell labeling, tissue repair, magnetic resonance imaging (MRI), and drug delivery [268,269]. Au NPs have proved to be important tool in many potential biomedical applications including an emerging alternative for life-threatening diseases and also have been used in DNA modeling [270,271]. Au NPs with different sizes display optical properties necessary for biosensor applications, especially in cancer nanotechnology. PEG coated Au NPs maximize the tumor damage as compared to Tumor necrosis factor-alpha (TNF-a), a cytokine which has anticancer efficacy, but limited therapeutic applications [272-274].
The use of nanotechnology in medicine offers some exciting possibilities. Some techniques are only imagined, while others are at various stages of testing, or actually being used today. Nanotechnology in medicine involves applications of nanoparticles currently under development, as well as longer range research that involves the use of manufactured nano-robots to make repairs at the cellular level (sometimes referred to as nanomedicine). Whatever you call it, the use of nanotechnology in the field of medicine could revolutionize the way we detect and treat damage to the human body and disease in the future, and many techniques only imagined a few years ago are making remarkable progress towards becoming realities. Being super paramagnetic in nature, iron and iron oxide NPs find extensive usage in biomedical applications such as cell labeling, tissue repair, magnetic resonance imaging (MRI), and drug delivery [268,269]. Au NPs have proved to be important tool in many potential biomedical applications including an emerging alternative for life-threatening diseases and also have been used in DNA modeling [270,271]. Au NPs with different sizes display optical properties necessary for biosensor applications, especially in cancer nanotechnology. PEG coated Au NPs maximize the tumor damage as compared to Tumor necrosis factor-alpha (TNF-a), a cytokine which has anticancer efficacy, but limited therapeutic applications [272-274].
Au NPs exhibit high antibacterial activity due to their small size and high surface area. These MNPs suppress the respiratory chain enzymes which are vital for the cell wall synthesis of bacteria thereby leading to death or static growth. Spherical Au NPs synthesized from protein extract of blue green alga, S. platensis have been reported to show inhibitory action against B. subtilis and S. aureu. Since, gram positive bacteria have thick peptidoglycan layer, NPs adhere to the membrane and break the bonds thereby entering inside the microorganism [275]. Figure 29 depicts the damage caused to bacterial membrane on treatment with Au NPs.
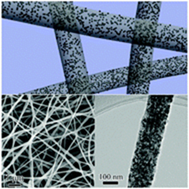
Figure 29: TEM images of (A) B. subtilis (B) S. aureus cells treated with S. platensis protein protected Au NPs in agar medium for 1h. Reproduced with permission [275].
Thus, functionalized NPs could be modified for use in advanced medical applications with greener methods. Banana peels rich in lignin, cellulose, pectins and hemicellulose act an excellent template for the synthesis of MNPs. The mechanism for the interaction of MNPs with the specific membrane has been illustrated in Figure 30. As soon as NP comes in contact with the membrane of pathogenic microorganisms, there is dissolution and release of metal cations which inhibit respiratory enzymes and ATP production. There is reactive oxygen species (ROS) production which disrupts membrane integrity and other transport processes [276].
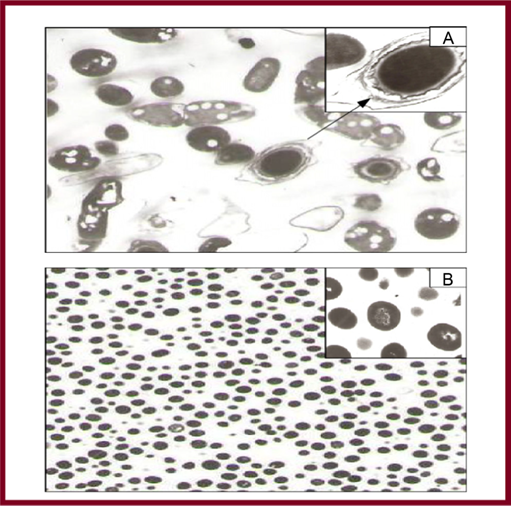
Figure 30: Representation of receptor-mediated uptake. This is the specific biological mechanism for particles interacting with the surface membrane and undergoing cellular uptake. The intrinsic NP characteristics that promote surface binding (roughness, hydrophobicity, cation charge) generally lead to nonspecific binding forces (marked by asterisks) that promote cellular uptake. In contrast, specific receptor–ligand interactions generally lead to endocytic uptake. A combination of nonspecific binding forces on the surface of spiked particles can lead to direct penetration of the surface membrane without the need to involve endocytic compartments. Reproduced with permission [276].
Various applications of MNPs are given in Figure 31. Antimicrobial activities of MNPs were well studied. Silver nanoparticles (AgNPs) and gold nanoparticles (AuNPs) have been reported to have a broad spectrum of antimicrobial activity against human and animal pathogens [277,278]. This is due to effective disruption of polymer subunits of cell membrane in a pathological organism, which in turn results in disturbance in bacterial system [279]. Membrane permeability of AgNPs can be increased by increasing the concentration and consequent rupture of cell wall can be achieved [280]. The interaction between silver and gold MNPs with cell membrane via binding to the active site is demonstrated [281]. Copper and copper oxide nanoparticles (CuNPs) were found to be effective antimicrobial agents [282]. CuNPs were found as strain specific antibacterial agents against Bacillus subtilis [283]. Anti-inflammatory activity of AgNPs was explored previously and it was due to inhibition of interferon-γ, tumor necrosis factor alpha (TNF-α); reducing matrix metallo proteinase (MMP) and proinflammatory cytokines [284,285]. Biosynthesized MNPs was proved to be having wound healing and tissue regeneration activity in inflammatory mechanism [286]. AgNPs were demonstrated to have antifungal activity [287] and fungicidal properties were explored against Candida sp. and results were found to be significant to that of fluconazole and amphotericin. This activity is may be due to damage to fungal intracellular components [288]. As commercially available antifungal products are had various side effects, developing and exploring multifunctional MNPs to combat fungal infections is recommended. AgNPs were active against malarial vectors and reported to have a larvicidal effect [289,290].
Green synthesized MNPs of silver, platinum, palladium are effective in controlling malarial population. There is an urgent need to search for an alternative against vectors that are responsible for spreading the most common plasmodial diseases [291,292]. Recently, many investigators reported that green synthesized MNPs have potential to control tumor cell growth. This activity is due to presence of secondary metabolites in plant extracts [293,294]. AgNPs and AuNPs were found to be effective against malignant cells [295]. AntiHIV activity of AgNPs was studied at an early stage of reverse transcription mechanism [296]. Biosynthesize MNPs significantly reduce the level of hepatic enzymes like alanine transaminase, alkaline phophatase, serum creatinine and uric acid in diabetes-induced mice [297]. AgNPs were potent inhibitors of α-amylase at 140 mg/dl [298,299]. Secondary metabolites present in plant derived MNPs are responsible for antioxidant activity [300].
MNPs in drug delivery
In recent years, interest has been generated in the capability of MNPs to bind a wide range of organic molecules, their low toxicity and their strong and tunable absorption. Unique chemical, physical and photo-physical properties of MNPs paved innovative ways in drug delivery systems to achieve controlled transport, controlled release and specific targeting of drugs [301-304]. It has been shown that conjugates of MNPs with antibiotics provide promising results in antimicrobial therapy [303]. Combination of antibiotic with MNPs would be helpful to improve antibiotic efficacy. This conjugation can be via covalent, ionic or physical absorption [305,306].
In recent years, interest has been generated in the capability of MNPs to bind a wide range of organic molecules, their low toxicity and their strong and tunable absorption. Unique chemical, physical and photo-physical properties of MNPs paved innovative ways in drug delivery systems to achieve controlled transport, controlled release and specific targeting of drugs [301-304]. It has been shown that conjugates of MNPs with antibiotics provide promising results in antimicrobial therapy [303]. Combination of antibiotic with MNPs would be helpful to improve antibiotic efficacy. This conjugation can be via covalent, ionic or physical absorption [305,306].
Cisplatin conjugation to MNPs has shown a significant cytotoxic effect, which is seven times higher than that of cisplatin alone. Conjugation of methotrexate to AuNPs, which involves the interaction of carboxylic group of drug with the metal surface found to have high concentrations of drug in Lewis lung carcinoma cells [307]. Conjugation of tamoxifen and AuNPs has been reported [308]. MNPs surfaces have to be modified in order to avoid aggregation and to improve the efficiency of MNPs drug delivery systems [309]. Doxorubicin in combination with surface modified AuNPs reported to have significant cytotoxicity when compared to the free form of doxorubicin [310]. Polyethylene glycol (PEG) can be used to modify the surface of MNPs so that the cellular uptake of nanoparticles can be improved [311]. AntiHER antibody-targeted gold/silicon nanoparticles in the form of nanoshells to treat metastatic breast cancer[312], aminosilane coated iron oxide nanoparticles to treat brain tumors[313] and starch coated iron oxide nanoparticles in the form of magnetically guided mitoxantrone to treat tumor angiogenesis[314] have been reported. Calcium phosphate nanoparticles as vaccine adjuvant (Biovant) is developed by Biosante for subcutaneous administration has entered into phase I trials [315].
Author previously reported a simple, cost effective and eco-friendly method for the synthesis of water soluble AgNPs using root bark extract of Azadirachta indica (RBAI). Clinical ultrasound gel prepared from these NPs proved to be effective with significant antibacterial activity [316]. One application of nanotechnology in medicine currently being developed involves employing nanoparticles to deliver drugs, heat, light or other substances to specific types of cells (such as cancer cells). Particles are engineered so that they are attracted to diseased cells, which allows direct treatment of those cells. This technique reduces damage to healthy cells in the body and allows for earlier detection of disease. For example, nanoparticles that [32].
(http://www.rsc.org/chemistryworld/News/2006/April/11040601.asp) deliver chemotherapy drugs directly to cancer cells (nanoparticle-chemotherapy.html) are under development. Tests are in progress for targeted delivery of chemotherapy drugs and their final approval for their use with cancer patients is pending. One company, CytImmune has published the results of a Phase 1 Clinical Trial (http://www.cytimmune.com/download/posters/ASCO_Poster.pdf#zone-clinical-wrapper) of their first targeted chemotherapy drug and another company, BIND Biosciences, has published preliminary results of a Phase 1 Clinical Trial (phase-%201-clinical-data-BIND-014.htm) for their first targeted chemotherapy drug and is proceeding with a Phase 2 Clinical Trial (clinical-trial-accurin-cancer.html).
Researchers at the Wyss Institue are testing nanoparticles that release drugs when subjected to sheer force, such as occurs when passing through a section of artery that is mostly blocked by a clot. Lab tests on animals have shown that this method is effective in delivering drugs used to dissolve clots. The Read more about their study here (clot_dissoloving_nanotherapeutic.html).
Researchers at the Houston Methodist Research Institute have demonstrated a targeted drug delivery method in mice using silicon nanoparticles (drug-delivery-nanoporous-silicon-polymer.html) that degrade inside a tumor, releasing polymer strands that form a nanoparticle containing the drug to be delivered. This polymer nanoparticle dissolves inside the cancer cell, delivering the drug to the cancer cell.
Researchers at the University of Illinois have demonstrated that gelatin nanoparticles (gelatin-nanoparticles-braindrug-delivery.html) can be used to deliver drugs to damaged brain tissue more efficiently than standard methods. This has been demonstrated in the lab, the researchers hope that this method will result in more effective drug delivery for brain injuries.
Researchers at MIT are investigating the use of nanoparticles to deliver vaccine (nanoparticle-vaccine.html).The nanoparticles protect the vaccine, allowing the vaccine time to trigger a stronger immune response as shown in lab tests with mice. Additional work needs to be done to adapt the technique to human patients.
Researchers are developing a method to release insulin that uses a sponge-like matrix that contains insulin as well as nanocapsules (insulin-release-nanocapsule.html) containing an enzyme. When the glucose level rises the nanocapsules release hydrogen ions, which bind to the fibers making up the matrix. The hydrogen ions make the fibers positively charged, repelling each other and creating openings in the matrix through which insulin is released. So far this has been shown to be effective in tests with lab mice.
Researchers are developing a nanoparticle that can be taken orally (nanoparticles-oral-drug-delivery.html) and pass through the lining of the intestines into the bloodsteam. This should allow drugs that must now be delivered with a shot to be taken in pill form. The researchers have demonstrated the technique with lab mice so far. Researchers are also developing a nanoparticle to defeat viruses. The nanoparticle does not actually destroy viruses’ molecules, but delivers an enzyme that prevents the reproduction of virus's molecules (nanozyme-viral-infections.html) in the patients’ bloodstream. The effectiveness of the technique has been demonstrated in lab tests. (Nanotechnology-drug-delivery.html).
Therapy Techniques
Researchers have developed "nanosponges" that absorb toxins and remove them from the bloodstream. The nanosponges (nanosponges-toxins.html) are polymer nanoparticles coated with a red blood cell membrane. The red blood cell membrane allows the nanosponges to travel freely in the bloodstream and attract the toxins. Researchers have demonstrated a method to generate sound waves that are powerful, but also tightly focused, that may eventually be used for noninvasive surgery. They use a lens coated with carbon nanotubes (nanotube-lens noninvasive- surgery.html) to convert light from a laser to focused sound waves. The intent is to develop a method that could blast tumors or other diseased areas without damaging healthy tissue.
Researchers have developed "nanosponges" that absorb toxins and remove them from the bloodstream. The nanosponges (nanosponges-toxins.html) are polymer nanoparticles coated with a red blood cell membrane. The red blood cell membrane allows the nanosponges to travel freely in the bloodstream and attract the toxins. Researchers have demonstrated a method to generate sound waves that are powerful, but also tightly focused, that may eventually be used for noninvasive surgery. They use a lens coated with carbon nanotubes (nanotube-lens noninvasive- surgery.html) to convert light from a laser to focused sound waves. The intent is to develop a method that could blast tumors or other diseased areas without damaging healthy tissue.
Researchers are investigating the use of bismuth nanoparticles (bismuth-nanoparticles-radiation-therapy.html) to concentrate radiation used in radiation therapy to treat cancer tumors. Initial results indicate that the bismuth nanoparticles would increase the radiation dose to the tumor by 90 percent. Nanoparticles composed of polyethylene glycol-hydrophilic carbon clusters (PEG-HCC) have been shown to absorb free radicals (nanoparticles-free-radicals-brain-injury.htm) at a much higher rate than the proteins out body uses for this function. This ability to absorb free radicals may reduce the harm that is caused by the release of free radicals after a brain injury.
Targeted heat therapy is being developed to destroy breast cancer tumors. In this method antibodies that are strongly attracted to proteins produced in one type of breast cancer cell are attached to nanotubes, causing the nanotubes to accumulate at the tumor. Infrared light from a laser is absorbed by the nanotubes and produces heat that incinerates the tumor (nanomedicine-nanotubes-breast-cancer.html). (Nanotechnology-therapy-techniques.html). Altogether, published literatures suggest that the AgNPs is a suitable promising agent to inhibit the growth of cancer cells via various mechanistic approaches. The hypothetical mechanism is shown in Figure 32.
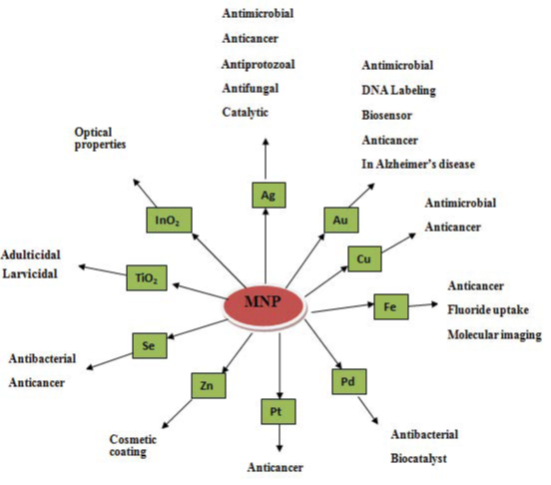
Figure 32: The possible mechanisms of AgNP-induced cytotoxicity in cancer cell lines. Endoplasmic reticulum stress(ER), lactate dehydrogenase (LDH), reactive oxygen species (ROS).
Diagnostic Techniques
Researchers at Worcester Polytechnic Institute are using antibodies attached to carbon nanotubes (nanotube-cancersensor.html) in chips to detect cancer cells in the blood stream. The researchers believe this method could be used in simple lab tests that could provide early detection of cancer cells in the bloodstream. Researchers at MIT have developed a sensor using carbon nanotubes embedded in a gel; that can be injected under the skin to monitor the level of nitric oxide (carbon-nanotubes-implant-sensor.html) in the bloodstream. The level of nitric oxide is important because it indicates inflammation, allowing easy monitoring of inflammatory diseases. In tests with laboratory mice the sensor remained functional for over a year.
Researchers at Worcester Polytechnic Institute are using antibodies attached to carbon nanotubes (nanotube-cancersensor.html) in chips to detect cancer cells in the blood stream. The researchers believe this method could be used in simple lab tests that could provide early detection of cancer cells in the bloodstream. Researchers at MIT have developed a sensor using carbon nanotubes embedded in a gel; that can be injected under the skin to monitor the level of nitric oxide (carbon-nanotubes-implant-sensor.html) in the bloodstream. The level of nitric oxide is important because it indicates inflammation, allowing easy monitoring of inflammatory diseases. In tests with laboratory mice the sensor remained functional for over a year.
Researchers at the University of Michigan are developing a sensor that can detect a very low level of cancer cells, as low as 3 to 5 cancer cells in a one milliliter in a blood sample. They grow sheets of graphene oxide (graphene-oxide cancer- cell-sensor.html), on which they attach molecules containing an antibody that attaches to the cancer cells. They then tag the cancer cells with fluorescent molecules to make the cancer cells stand out in a microscope. Researchers have demonstrated a way to use nanoparticles for early diagnosis of infectious disease (nanoparticleinfection-early-detection.html). The nanoparticles attach to molecules in the blood stream indicating the start of an infection. When the sample is scanned for Raman scattering the nanoparticles enhance the Raman signal, allowing detection of the molecules indicating an infectious disease at a very early stage.
A test for early detection of kidney damage is being developed. The method uses gold nanorods (gold-nanorod-kidney function- test.html) functionalized to attach to the type of protein generated by damaged kidneys. When protein accumulates on the nanorod the color of the nanorod shifts. The test is designed to be done quickly and inexpensively for early detection of a problem. (Nanotechnology-medical-diagnosis.html).
Anti-Microbial Techniques
In recent years, increasing antibiotic resistance by microbes is imposing serious threat to the health sector. Nanoparticles have proved to be a likely candidate for antimicrobial agent since their large surface to volume ratio ensures a broad range of attack on bacterial surface. Researchers at the University of Houston are developing a technique to kill bacteria using gold nanoparticles and infrared light (gold-nanoparticles-bacteria.html). This method may lead to improved cleaning of instruments in hospital settings.
In recent years, increasing antibiotic resistance by microbes is imposing serious threat to the health sector. Nanoparticles have proved to be a likely candidate for antimicrobial agent since their large surface to volume ratio ensures a broad range of attack on bacterial surface. Researchers at the University of Houston are developing a technique to kill bacteria using gold nanoparticles and infrared light (gold-nanoparticles-bacteria.html). This method may lead to improved cleaning of instruments in hospital settings.
Researchers at the University of Colorado Boulder are investigating the use of quantum dots (quantum-dots-antibiotic resistant- infections.html) to treat antibiotic resistant infections. Researchers at the University of New South Wales are investigating the use of polymer coated iron oxide nanoparticles (iron-oxide-nanoparticles-bacteria.html) to treat chronic bacterial infections. One of the earliest nanomedicine applications was the use of nanocrystalline silver (http://www.cesil.com/leaderforchemist/articoli/inglese/7demlinging/7demlinging.htm) which is as an antimicrobial agent for the treatment of wounds, as discussed on the Nucryst Pharmaceuticals Corporation (http://www.nucryst.com/acticoat_dressings.htm) website.
A nanoparticle cream has been shown to fight staph infections. The nanoparticles contain nitric oxide gas (nanomedicine-nanoparticles-cream-no-staph.html), which is known to kill bacteria. Studies on mice have shown that using the nanoparticle cream to release nitric oxide gas at the site of staph abscesses significantly reduced the infection. Burn dressing that is coated with nanocapsules containing antibotics (nanocapsules-release-antibotics.html). If an infection starts the harmful bacteria in the wound causes the nanocapsules to break open, releasing the antibiotics. This allows much quicker treatment of an infection and reduces the number of times a dressing has to be changed.
A welcome idea in the early study stages is the elimination of bacterial infections (http://www.foresight.org/Nanomedicine/SayAh/index.html) [317] in a patient within minutes, instead of delivering treatment with antibiotics over a period of weeks. You can read about design analysis for the antimicrobial nanorobot used in such treatments in the following article: Microbivores: Artifical Mechanical Phagocytes using Digest and Discharge Protocol (http://www.rfreitas.com/Nano/Microbivores.htm) [318].
Anti-bactericidal activities of metallic nanoparticles
The reciprocal action of nanoparticles subsequently breaks the cell membrane and disturbs the protein synthesis mechanism in the bacterial system [319]. The interactions of bacteria and the metallic silver and gold nanoparticles have been binding with active site of cell membrane to inhibit the cell cycle functions [61].
The reciprocal action of nanoparticles subsequently breaks the cell membrane and disturbs the protein synthesis mechanism in the bacterial system [319]. The interactions of bacteria and the metallic silver and gold nanoparticles have been binding with active site of cell membrane to inhibit the cell cycle functions [61].
Anti-fungicidal activities of metallic nanoparticles
The fungicidal mechanism of biosynthesized metallic nanoparticles has more potential than commercial antibiotics such as fluconazole and amphotericin. Most of the commercial antifungal agents have limited applications clinically and in addition, there are more adverse effect and less recovery from the microbial disease. Subsequently, the commercial drugs induce side effect such as renal failure, increased body temperature, nausea, liver damage, and diarrhoea after using the drugs. Nanoparticles were developed for novel and effective drug against microbes. The fungal cell wall is made up of high polymer of fatty acid and protein. The fungal cell membrane structure significant changes were observed by treating it with metallic nanoparticles [320].
The fungicidal mechanism of biosynthesized metallic nanoparticles has more potential than commercial antibiotics such as fluconazole and amphotericin. Most of the commercial antifungal agents have limited applications clinically and in addition, there are more adverse effect and less recovery from the microbial disease. Subsequently, the commercial drugs induce side effect such as renal failure, increased body temperature, nausea, liver damage, and diarrhoea after using the drugs. Nanoparticles were developed for novel and effective drug against microbes. The fungal cell wall is made up of high polymer of fatty acid and protein. The fungal cell membrane structure significant changes were observed by treating it with metallic nanoparticles [320].
Antiplasmodial activity of metallic nanoparticles
Currently, the most common diseases are spreading everywhere by vectors. Vector control is a serious requirement in epidemic disease situation. The advanced antiplasmodial species specific control method is less effective. This method has been more economical but less effective to control the target organisms in the health care sector [321]. Hence, effective and affordable antimalarial drugs are urgently required to control the plasmoidal activity. In last few decades, plants have been used as traditional sources of natural products and having enough sources for drug development for antimalarial disease.
Currently, the most common diseases are spreading everywhere by vectors. Vector control is a serious requirement in epidemic disease situation. The advanced antiplasmodial species specific control method is less effective. This method has been more economical but less effective to control the target organisms in the health care sector [321]. Hence, effective and affordable antimalarial drugs are urgently required to control the plasmoidal activity. In last few decades, plants have been used as traditional sources of natural products and having enough sources for drug development for antimalarial disease.
The plant derived chemical constituents such as quinine, artemisinin and aromatic compound have been successfully used against resistant strains of malaria parasites [322]. Due to that high resistance of parasites, the alternative drug is needed for controlling the resistance strains. The plants developed metallic nanoparticles such as silver, platinum and palladium nanoparticles are effectively control the malarial population in the environment. Also, the biogenic synthesis of metallic silver nanoparticles from plant extract have been used to suppress the number of malarial productions.
Anti-inflammatory action of nanoparticles
Anti-inflammatory is an important wound healing mechanism. Anti-inflammation is a cascade process that produces immune responsive compound such as interleukins and cytokinins which can be produced by keratinocytes including T lymphocytes, B lymphocytes and macrophages [323]. Various inflammatory mediators such as enzymes, antibodies are secreted from the endocrine system. Other potential anti-inflammatory agents such as cytokines, IL-1, IL-2 are secreted from the primary immune organs. These anti-inflammatory mediators induce the healing process [324]. Also, the inflammatory mediators are involved in biochemical pathways and control the expansion of diseases. Biosynthesized gold nanoparticles achieved positive wound repair mechanisms and tissue regeneration in inflammatory function [325]. The studies proved that biosynthesized gold and platinum nanoparticles are alternative sources for treating inflammation in a natural way.
Anti-inflammatory is an important wound healing mechanism. Anti-inflammation is a cascade process that produces immune responsive compound such as interleukins and cytokinins which can be produced by keratinocytes including T lymphocytes, B lymphocytes and macrophages [323]. Various inflammatory mediators such as enzymes, antibodies are secreted from the endocrine system. Other potential anti-inflammatory agents such as cytokines, IL-1, IL-2 are secreted from the primary immune organs. These anti-inflammatory mediators induce the healing process [324]. Also, the inflammatory mediators are involved in biochemical pathways and control the expansion of diseases. Biosynthesized gold nanoparticles achieved positive wound repair mechanisms and tissue regeneration in inflammatory function [325]. The studies proved that biosynthesized gold and platinum nanoparticles are alternative sources for treating inflammation in a natural way.
Anticancer studies on plant mediated nanoparticles
Cancer is an uncontrolled cell proliferation with hysterical changes of biochemical and enzymatic parameters, which is universal property of tumour cells. The overexpression of cellular growth will be arrested and regulated with systematic cell cycle mechanisms in cancerous cell by using bio-based nanoparticles as novel controlling agents [115]. Also the plant mediated nanoparticles have great effect against various cancer cell lines such as Hep 2, HCT 116 and Hela cell lines. Recently, many studies reported that plant derived nanoparticles have potential to control tumour cell growth. The improved cytotoxic effect is due to the secondary metabolites and other non-metal composition in the synthesizing medium [326,327]. The plant derived silver nanoparticles regulate the cell cycle and enzymes in bloodstream [328]. Moreover, the plant synthesized nanoparticles relatively control the free radicals formation from the cell. Free radicals commonly induce cell proliferation and damage the normal cell function.
Cancer is an uncontrolled cell proliferation with hysterical changes of biochemical and enzymatic parameters, which is universal property of tumour cells. The overexpression of cellular growth will be arrested and regulated with systematic cell cycle mechanisms in cancerous cell by using bio-based nanoparticles as novel controlling agents [115]. Also the plant mediated nanoparticles have great effect against various cancer cell lines such as Hep 2, HCT 116 and Hela cell lines. Recently, many studies reported that plant derived nanoparticles have potential to control tumour cell growth. The improved cytotoxic effect is due to the secondary metabolites and other non-metal composition in the synthesizing medium [326,327]. The plant derived silver nanoparticles regulate the cell cycle and enzymes in bloodstream [328]. Moreover, the plant synthesized nanoparticles relatively control the free radicals formation from the cell. Free radicals commonly induce cell proliferation and damage the normal cell function.
The moderate concentration of gold nanoparticles induces the apoptosis mechanism in malignant cells [329]. Similarly, Ag nanoparticles treated MCF-7 cancer cell line has retained the biomolecules concentration in the cells, and subsequently the cell metabolism was regulated [154]. The metallic nanoparticles have proved their novel applications in medical field to diagnose and treat various types of cancer and other retroviral diseases. The biobased nanoparticles are new and revolutionized to treat malignant deposit and without interfering the normal cells. Suman., et al. (2013) [330] reported that the green synthesis of silver nanoparticles exhibited a significant cytotoxic effect in HeLa cell lines compared to other chemical based synthetic drugs.
Antiviral effects of metallic nanoparticles
Plants mediated nanoparticles are the alternative drugs for treating and controlling the growth of viral pathogens. The entry of viruses into a host is very reckless and it involved in faster translational process to multiply their colony numbers. The metallic MNPs are strong antiviral agents and inhibit the viral entry into the host system. The biosynthesized metallic nanoparticles have multiple binding sites to bind with gp120 of viral membrane to control the function of virus. The bio-based nanoparticles are acting as effective virucidal agent against cell-free virus and cell-associated virus [331]. In addition, the silver and gold nanoparticles are constantly inhibiting post-entry stages of the HIV-1 life cycle. Therefore, the metallic nanoparticles will act as promising antiviral drug against retro viruses.
Plants mediated nanoparticles are the alternative drugs for treating and controlling the growth of viral pathogens. The entry of viruses into a host is very reckless and it involved in faster translational process to multiply their colony numbers. The metallic MNPs are strong antiviral agents and inhibit the viral entry into the host system. The biosynthesized metallic nanoparticles have multiple binding sites to bind with gp120 of viral membrane to control the function of virus. The bio-based nanoparticles are acting as effective virucidal agent against cell-free virus and cell-associated virus [331]. In addition, the silver and gold nanoparticles are constantly inhibiting post-entry stages of the HIV-1 life cycle. Therefore, the metallic nanoparticles will act as promising antiviral drug against retro viruses.
Antidiabetic management of metallic nanoparticles
Diabetes Mellitus (DM) is a group of metabolic dysfunction in which person has uncontrolled sugar level in blood. Certain foods and balance diet or synthetic insulin drugs can be prevent the diabetes at certain levels, but the complete treatment of DM is a big challenge. However, the biosynthesized nanomaterials could be alternative drug to cure the diabetes mellitus. Daisy and Saipriya’s (2012) [332] results showed that gold nanoparticles have good therapeutic effects against diabetic models. Gold nanoparticles significantly reduce the level of liver enzymes such as alanine transaminase, alkaline phosphatase, serum creatinine, and uric acid in treated diabetes mice.
Diabetes Mellitus (DM) is a group of metabolic dysfunction in which person has uncontrolled sugar level in blood. Certain foods and balance diet or synthetic insulin drugs can be prevent the diabetes at certain levels, but the complete treatment of DM is a big challenge. However, the biosynthesized nanomaterials could be alternative drug to cure the diabetes mellitus. Daisy and Saipriya’s (2012) [332] results showed that gold nanoparticles have good therapeutic effects against diabetic models. Gold nanoparticles significantly reduce the level of liver enzymes such as alanine transaminase, alkaline phosphatase, serum creatinine, and uric acid in treated diabetes mice.
The gold nanoparticles treated diabetic model showed a decrease of HbA (glycosylated haemoglobin) level which is maintaining the normal range [333] explored the Sphaeranthus amaranthoides biosynthesized silver nanoparticles inhibited a-amylase and acarbose sugar in diabetes induced animal model. It is mainly a-amylase inhibitory components are present in ethanolic extract of S. amaranthoides [334]. Likewise, [335] studied that the nanoparticles are potent therapeutic agent to control diabetes with few side effects. The clinical studies in mice successfully control the sugar level of 140 mg/dl in silver nanoparticles treated group.
Antioxidant mechanisms of plant derived nanoparticles
Antioxidant agents including enzymatic and non-enzymatic substances regulate the free radical formation. Free radicals are causing cellular damage including brain damage, atherosclerosis and cancer. The free radicals are generated by reactive oxygen species (ROS) such as superoxide dismutase, hydrogen peroxides and hydrogen radicals. Biomolecules such as proteins, glycoprotein, lipids, fatty acids, phenolics, flavonoids and sugars strongly controlled the free radical formation [336]. The scavenging power of enzymatic and non-enzymatic antioxidants is useful for the management of various chronic diseases such as diabetes, cancer, AIDS, nephritis, metabolic disorders and neurodegenerative. The antioxidant effect of silver nanoparticles was stronger than other synthetic commercial standards e.g. ascorbic acid and so on. The nanoparticles showed higher antioxidant activity whereas the tea leaf extract possesses higher phenolic and flavonoids content in the extract [337].
Antioxidant agents including enzymatic and non-enzymatic substances regulate the free radical formation. Free radicals are causing cellular damage including brain damage, atherosclerosis and cancer. The free radicals are generated by reactive oxygen species (ROS) such as superoxide dismutase, hydrogen peroxides and hydrogen radicals. Biomolecules such as proteins, glycoprotein, lipids, fatty acids, phenolics, flavonoids and sugars strongly controlled the free radical formation [336]. The scavenging power of enzymatic and non-enzymatic antioxidants is useful for the management of various chronic diseases such as diabetes, cancer, AIDS, nephritis, metabolic disorders and neurodegenerative. The antioxidant effect of silver nanoparticles was stronger than other synthetic commercial standards e.g. ascorbic acid and so on. The nanoparticles showed higher antioxidant activity whereas the tea leaf extract possesses higher phenolic and flavonoids content in the extract [337].
Cell Repair
Nanorobots could actually be programmed to repair specific diseased cells, functioning in a similar way to antibodies in our natural healing processes. Read about design analysis for one such cell repair nanorobot in this article: The Ideal Gene Delivery Vector: Chromallocytes, Cell Repair Nanorobots for Chromosome Repair Therapy (http://jetpress.org/v16/freitas.pdf).
Nanorobots could actually be programmed to repair specific diseased cells, functioning in a similar way to antibodies in our natural healing processes. Read about design analysis for one such cell repair nanorobot in this article: The Ideal Gene Delivery Vector: Chromallocytes, Cell Repair Nanorobots for Chromosome Repair Therapy (http://jetpress.org/v16/freitas.pdf).
Resources
National Cancer Institute Alliance for Nanotechnology in Cancer; this alliance includes a Nanotechnology Characterization Lab (http://ncl.cancer.gov/) as well as eight Centers of Cancer Nanotechnology Excellence (http://nano.cancer.gov/action/programs/ccne.asp). Alliance for NanoHealth (http://www.nanohealthalliance.org/); this alliance includes eight research institutions performing collaborative research. European Nanomedicine platform (http://www.etp-nanomedicine.eu/public).The National Institute of Health (NIH) is funding research at eight Nanomedicine Development Centers (http://nihroadmap.nih.gov/nanomedicine/fundedresearch.asp). Nanomedicine based upon nano-robots (nanomedicine.html).
National Cancer Institute Alliance for Nanotechnology in Cancer; this alliance includes a Nanotechnology Characterization Lab (http://ncl.cancer.gov/) as well as eight Centers of Cancer Nanotechnology Excellence (http://nano.cancer.gov/action/programs/ccne.asp). Alliance for NanoHealth (http://www.nanohealthalliance.org/); this alliance includes eight research institutions performing collaborative research. European Nanomedicine platform (http://www.etp-nanomedicine.eu/public).The National Institute of Health (NIH) is funding research at eight Nanomedicine Development Centers (http://nihroadmap.nih.gov/nanomedicine/fundedresearch.asp). Nanomedicine based upon nano-robots (nanomedicine.html).
Environmental aspects of the use of nanoparticles
The growth of population and urbanization along with poor water supply and environmental hygiene are the main reasons for the increase in outbreak of infectious pathogens. Transmission of infectious pathogens to the community has caused outbreaks of diseases such as influenza (A/H5N1), diarrhea (Escherichia coli), cholera (Vibrio cholera), etc. throughout the world. The comprehensive treatments of environments containing infectious pathogens using advanced disinfectant nanomaterials and nanoparticles have been proposed for prevention of the outbreaks. In recent years, the outbreak of re-emerging and emerging infectious diseases has been a significant burden on global economies and public health.
The growth of population and urbanization along with poor water supply and environmental hygiene are the main reasons for the increase in outbreak of infectious pathogens. Transmission of infectious pathogens to the community has caused outbreaks of diseases such as influenza (A/H5N1), diarrhea (Escherichia coli), cholera (Vibrio cholera), etc. throughout the world. The comprehensive treatments of environments containing infectious pathogens using advanced disinfectant nanomaterials and nanoparticles have been proposed for prevention of the outbreaks. In recent years, the outbreak of re-emerging and emerging infectious diseases has been a significant burden on global economies and public health.
Global demand for nanomaterials increases year by year. It is related with inevitable penetration of nanoparticles into the environment. It may have a substantial impact on the functioning of living organisms. The Commission to the European Parliament, the Council and the European Economic and Social Committee claims that monitoring the presence of nanoparticles in the environment is a major technical challenge. The small size of nanomaterials, and their low, and sometimes trace level, abundance in environmental samples is the main problem. Despite the fact that science has some methods of controlling environmental pollution by nanomaterials, the current state of development assumes that they are not fully verified. That makes it difficult to compare the available data and does not allow for a thorough analysis of the problem [240].
There is no doubt that the presence of nanoparticles in many consumer products generally improves the quality of life, providing numerous benefits and economic utility. The nanoparticles have been introduced in the formulation of different types of products. Because of the nanometric particles size, one may not observe their chemical and physical effects on living matter [338]. Penetration of nanomaterials into environment and its specific reactivity can cause degradation effects. Therefore, there is a need to develop techniques to monitor potential risks [339].
The impact of nanoparticles on the environment can take many forms. It is worth noting that weather factors such as temperature or humidity can also affect the physical properties of nanoparticles (size and stability). These processes are lengthy, but one should be aware that nanoparticles once introduced into the environment affect pollution of soil, surface water and groundwater as well as flora and fauna. Living organisms may be not exposed to nanomaterials during their life, but toxicological concerns can be a problem in the future when the processes of penetration of nanomaterials reach the stage of direct contact with successive generations of living matter [30].
Mechanisms of toxic effect of nanoparticles on organisms
Mostly, the toxicity of the product is determined as a function of its mass. In the case of nanomaterials this assumption is incorrect and one must use a different technique for risk assessment. Nanoparticles can penetrate into living organisms by means of swallowing, inhalation, absorption and penetration through the skin. Understanding the life cycle of nanoparticles in the environment and their chemical stability is an important step in the process of determining their influence on living organisms. Despite the lack of thorough research, a framework to conduct analysis has been developed. It involves:
Mostly, the toxicity of the product is determined as a function of its mass. In the case of nanomaterials this assumption is incorrect and one must use a different technique for risk assessment. Nanoparticles can penetrate into living organisms by means of swallowing, inhalation, absorption and penetration through the skin. Understanding the life cycle of nanoparticles in the environment and their chemical stability is an important step in the process of determining their influence on living organisms. Despite the lack of thorough research, a framework to conduct analysis has been developed. It involves:
- Physicochemical characteristics of particles which may have a negative effect on living matter,
- Familiarity with the life cycle of nanomaterials and the mode of their penetration into living organisms,
- Selection of appropriate techniques to measure the degree of an organism’s exposure to the nanomaterials,
- defining the rules governing deployment of nanoparticles in different parts of living organisms,
- developing mechanisms for inducing conditions [30].
In the literature one can find two main hypotheses explaining the toxic effects of nanoparticles on living organisms [340]. The first hypothesis that the harmful activities of nanoparticles is due to the release of metal ions [340,341]. The second states that the toxicity is induced by formation of ROS (reactive oxygen species). The resulting free radicals are able to damage any components of the cell, and initiate the production of ever-increasing numbers of reactive oxygen species [342]. For example, generated free radicals are able to oxidize the double bonds of fatty acids in cell membranes, resulting in increased permeability of membranes, and increased permeability contributes to the osmotic stress [343]. ROS may also inhibit the activity of enzymes by binding to them and changing the helix of DNA, which can lead to cell death. The formation of larger amounts of reactive oxygen species is induced by the higher surface area of nanoparticles as compared to their larger analogues [343].
Nanomaterials may also damage the cell membrane, oxidize proteins, and be genotoxic as well as interfere with the conduction of energy [342]. Balancing the risks and benefits of nanomaterials is essential in carrying out safe and responsible studies of their development. There is a general belief that research on the toxicity of nanomaterials are lagging behind the desire for their commercial use [344]. There is an urgent need to standardize safety evaluation of nanomaterials, which would facilitate the assessment of exposure to nanomaterials and risks arising from their use. The aim of such an action is a safe assimilation of nanotechnology in society. Environmental organizations and public opinion treat silver nanoparticles as a key factor in campaign against the use of nanomaterials [53].
Drawbacks of MNPs
Few of the disadvantages of MNPs are the exact mechanism for synthesis of NPs needs to be elucidated, limitations to scale up production processes and reproducibility of the processes [345]. Chronic exposure to silver NPs causes adverse effects like argyria and argyrosis, soluble silver may cause organ damage [346]. Microbes are regarded as potential biofactories for MNPs synthesis and serve a new generation antimicrobial agents with their unique physicochemical properties. The MNPs have found diverse applications in the field of pharmacy as direct therapeutic agents to treat ailments and also as carriers for drug delivery systems. In both the cases, stability and surface activity of MNPs are the vital areas where researchers have to concentrate. In particular, the development of rational protocol for green synthesis of MNPs in keeping view of the advantages of this approach; application of these particles to resolve problem of antibiotic resistance and in the target specific drug delivery systems to treat microbial infections including HIV and cancer should be highly focused in the future.
Few of the disadvantages of MNPs are the exact mechanism for synthesis of NPs needs to be elucidated, limitations to scale up production processes and reproducibility of the processes [345]. Chronic exposure to silver NPs causes adverse effects like argyria and argyrosis, soluble silver may cause organ damage [346]. Microbes are regarded as potential biofactories for MNPs synthesis and serve a new generation antimicrobial agents with their unique physicochemical properties. The MNPs have found diverse applications in the field of pharmacy as direct therapeutic agents to treat ailments and also as carriers for drug delivery systems. In both the cases, stability and surface activity of MNPs are the vital areas where researchers have to concentrate. In particular, the development of rational protocol for green synthesis of MNPs in keeping view of the advantages of this approach; application of these particles to resolve problem of antibiotic resistance and in the target specific drug delivery systems to treat microbial infections including HIV and cancer should be highly focused in the future.
Conclusions
The potential of using metal nanoparticles MNPs in various fields increases the need to produce them on an industrial scale and in stable formulations with environmentally friendly processes. Therefore, much effort is being made towards exploiting natural resources and implementing biological synthesis methods with proven advantages, such as being environmentally friendly, easy to scale up, and cost-effective; thus, the green production of nanoparticles using biological resources has great potential. The biological route of synthesizing nanoparticles has many advantages, such as the stable production of nanoparticles with controlled sizes and shapes, the lack of subsequent complex chemical synthesis, the lack of toxic contaminants, and the ability for rapid synthesis using numerous medicinal plants and microorganisms. Green biological synthesis of MNPs using plant derivatives and different macro–microscopic organisms such as plant, bacteria, algae, fungi, seaweeds and microalgae is extremely studied. It is important to understand how active groups from biological sources attach to the nanoparticle surface, and which active groups are involved, to produce nanoparticles with higher efficacy. Thus, the plethora of microorganisms and plants that have been successfully used for the biological synthesis of metal nanoparticles prompts the deeper exploration of biological nanofactories to meet the need for nanoproducts in various fields. However, issues relating to the biomedical applications of biological nanoparticles, including the distribution profile, excretion, and clearance of nanoparticles in in vivo trials, need to be addressed. The plant derived metallic nanoparticles have projected impact on diagnosis and treatment of various diseases with controlled side effects. The up-and coming researches have proven their antimicrobial significance. Additionally, investigations into the biocompatibility and bioavailability of nanoparticles are still at early stages, and considerable research is needed in this direction. MNPs were developed for novel and effective drug against microbes, have a promising activity against spore producing fungus and effectively destroy the fungal growth. Recent advances in nanoscience and NPs nanotechnology radically changed the way we diagnose, treat, and prevent various diseases in all aspects of human life.
References
- Rao PV., et al. “Recent advances in nanotechnology- based diagnosis and treatments of diabetes”. Current Drug Metabolism 16.5 (2015): 371-375.
- Rai M., et al. “Strategic role of selected noble metal nano-particles in medicine”. Critical Reviews in Microbiology 42.5 (2015): 696-719.
- Abbasi E., et al. “Silver nanoparticles: synthesis methods, bio-applications and properties”. Critical Reviews in Microbiology 42.2 (2015): 170-180.
- Giljohann D A., et al. “Gold nanoparticles for biology and medicine”. Angewandte Chemie International Edition in English 49.19 (2010): 3280-3294.
- Pereira L., et al. “Metallic nanoparticles: microbial synthesis and unique properties for biotechnological applications, bioavail-ability and biotransformation”.Critical Reviews in Biotechnology 35.1 (2015): 114-128
- Madkour LH. “Vision for life sciences: interfaces between nanoelectronic and biological systems”. Global Drugs and Therapeutics 2.4 (2017): 1-4.
- Madkour LH. “Advanced AuNMs as Nanomedicine’scentral Goals Capable of Active Targeting in Both Imaging and Therapy in Biomolecules”. BAOJ Nanotechnology 3.1 (2017): 15-18.
- Madkour LH. “Biotechnology of Nucleic Acids Medicines as Gene Therapeutics and Their Drug Complexes”. Chronicles of Pharmaceutical Science 1.4 (2017): 204-253.
- Moore MN. “Do nanoparticles present ecotoxicological risks for the health of the aquatic environment?” Environment International 32.8 (2006): 967-976.
- Sergeev GB and Shabatina TI.” Cryochemistry of nanometals. Colloids and Surfaces A: Physicochemical and Engineering Aspects “(2008).
- Chenming Xue., et al. “Size- and Shape-Dependent Fluorescence Quenching of Gold Nanoparticles on Perylene Dye”. Advanced Optical Materials 1.8 (2013):581-587.
- Loai Aljerf and Rim Nadra. “Developed greener method based on MW implementation in manufacturing CNFs”. International Journal of Nanomanufacturing (2018).
- Toshiyuki Nomura., et al. “Shape and size control of barium chromate nanoparticles using reverse micelle”. Advanced Powder Technology 20.1 (2009): 101-105.
- Jyoti Katyal and R.K. Soni. “Size- and shape-dependent plasmonic properties of aluminum nanoparticles for nanosensing applications”. Journal of Modern Optics 60.20 (2013): 1717-1728.
- Taniguchi N. “On the basic concept of nanotechnology. In: Proc. of International Conference on Precision Engineering (ICPE)” Tokyo Japan (1974): 18-23.
- JSA Bhat. “Heralding a new future – Nanotechnology”. Current Science 85.2 (2003): 147-154.
- MTBohr. “Nanotechnology goals and challenges for electronic applications”. IEEE Transactions on Nanotechnology 99.1 (2002): 56-62.
- EM James. “Practical aspects of atomic resolution imaging and analysis in STEM”. Ultramicroscopy 78.1 (1999): 125-139.
- Sanjeeb KS and Vinod L. “Nanotech approaches to drug delivery and imaging”. Drug Discovery Today 8.24 (2003): 1112-1120.
- Kaushik N., et al. “Biological synthesis of metallic nanoparticles”. Nanomedicine: Nanotechnology, Biology and Medicine 6.2 (2010): 257-262.
- Kouvaris P., et al. “Green synthesis and characterization of silver nanoparticles produced using Arbutus unedo leaf extract”. Materials Letters 76.1 (2012): 18-20.
- Shameli K., et al. “Green biosynthesis of silver nanoparticles using Callicarpa maingayi stem bark extraction”. Molecules 17.7 (2012): 8506-8517.
- Parveen S., et al. “Nanoparticles: a boom to drug delivery, therapeutics, diagnostics and imaging”. Nanomedicine 8.2 (2012): 147-166.
- Gurunathan S., et al. “Purification and characterization of silver nanoparticles using Escherichia coli”. Colloids and Surfaces 74.1 (2009): 328-335.
- Hussain S and Pal A.K. “Incorporation of nanocrystalline silver on carbon nanotubes by electrodeposition technique”. Materials Letters 62.12 (2008): 1874-1877.
- Ahmad A., et al. “Extracellular biosynthesis of silver nanoparticles using the fungus Fusarium oxysporum”. Colloids and Surfaces 28.4 (2003): 313-318.
- Hill WR., et al. “Argyria: The Pharmacology of Silver”. (1939): 128-132.
- Lue JT. “A review characterization and physical property studies of metallic nanoparticles”. Journal of Physics and Chemistry of Solids 62.9 (2001): 1599-1612.
- Rai M., et al. “Silver nanoparticles as a new generation of antimicrobials”. Biotechnology Advances 27.1 (2009): 76-83.
- Lu K. “Nanoparticulate materials. Synthesis, characterization, and processing”. (2013).
- Porter A and Youtie J. “How interdisciplinary is nanotechnology”. Journal of Nanoparticle Research 11.5 (2009): 1023-1041.
- Jon Evans., et al.” Nanotechnology tackles chemotherapy”. Proceedings of the National Academy of Sciences (2006).
- Michael Berger. “Carbon nanotubes could make t-shirts bullet proof”. Nanotechnology Spotlight (2006).
- Ju-Nam Y and Lead J R. Science of the Total Environment 1: 400.
- De M Ghosh P S and Rotello V M. Advanced Materials 20 (2008): 4225.
- Lu A-H Salabas E L and Ferdi Sch¨uth Angewandte. Chemie International Edition 46 (2007): 1222.
- Ghosh Chaudhuri R and Paria S Chemical Reviews. 112 (2012): 2373.
- Abou El-Nour., et al. “Synthesis and applications of silver nanoparticles” Arabian Journal of Chemistry 3.3 (2010): 135-140.
- Mohanpuria P., et al. “Biosynthesis of nanoparticles: technological concepts and future applications”. Journal of Nanoparticle Research 10.3 (2008): 507-517.
- Poulose S., et al. “Biosynthesis of silver nanoparticles.” Journal of Nanoscience and Nanotechnology 14.2 (2014): 2038-2049.
- Vijayakumar., et al. “Biosynthesis, characterisation and anti-bacterial effect of plant-mediated silver nanoparticles using Artemisia nilagirica.” Industrial Crops and Products 41.1 (2013): 235-240.
- Parsons JG., et al. “Chapter 21 Use of plants in biotechnology: Synthesis of metal nanoparticles by inactivated plant tissues, plant extracts, and living plants”. Developments in Environmental Science 5 (2007): 463-485.
- Antonyraj CA., et al. “Selective oxidation of HMF to DFF using Ru/calumina catalyst in moderate boiling solvents toward industrial production”. Journal of Industrial and Engineering Chemistry 19 .3 (2013): 1056-1059.
- Frances N., et al. “Novel one-pot synthesis and characterization of bioactive thiol-silicate nanoparticles for biocatalytic and biosensor applications”. Nanotechnology 20 .5 (2009): 055612.
- Staniland SS. “Magnetosomes: Bacterial Biosynthesis of Magnetic Nanoparticles and Potential Biomedical Applications”. Nanotechnologies for the Life Sciences (2007).
- Fu H., et al. “Bimetallic Ag–Au nanowires: synthesis, growth mechanism, and catalytic properties”. Langmuir 29.23 (2013): 7134-7142.
- Kwon SJ and Bard AJ. “DNA analysis by application of Pt nanoparticle electrochemical amplification with single label response”. Journal of the American Chemical Society 134 .26 (2012): 10777-10779.
- Roy N., et al. “Green synthesis of silver nanoparticles: an approach to overcome toxicity”. Environmental Toxicology and Pharmacology 36.3 (2013): 807-812.
- Luo X., et al. “Application of nanoparticles in electrochemical sensors and biosensors”. Electroanalysis 18.4 (2006): 319-326.
- Murthy SK. “Nanoparticles in modern medicine: State of the art and future challenges”. International Journal of Nanomedicine 2.2 (2007): 129-141.
Wen ZQ., et al. “Detection of trace melamine in raw materials used for protein pharmaceutical manufacturing using surface-enhanced Raman spectroscopy (SERS) with gold nanoparticles”. Applied Spectroscopy 65.5 (2011): 514-521. - Xia Y., et al. “Nanoparticles for catalysis”. Accounts of Chemical Research 46.8 (2013): 1671-1672.
- Nanomaterials, The global market, Forecast from 2010 to 2025, Future markets, Edition. 2, February (2015).
- Commission recommendation on the definition of nanomaterial (2011/696/EU), Official Journal of the European Union, L 275/38, and 20.10. (2011).
- Chandran SP., et al. “Synthesis of gold nanotriangles and silver nanoparticles using Aloe vera plant extract”. Biotechnology Progress 22.2 577-583.
- Song JY and Kim BS. “Biological synthesis of bimetallic Au/Ag nanoparticles using Persimmon (Diospyros kaki) leaf extract”. Korean Journal of Chemical Engineering 25 (2009): 808-811.
- Monda S., et al. “Biogenic synthesis of Ag, Au and bimetallic Au/Ag alloy nanoparticles using aqueous extract of mahogany (Swietenia mahogani JACQ.) leaves.” Colloids and Surfaces B: Biointerfaces 82.2 (2011): 497-504.
- Bar H., et al. “Green synthesis of silver nanoparticles using latex of Jatropha curcas”. Colloids and Surfaces A: Physicochemical and Engineering Aspects 339.1-3 (2009): 134-139.
- Cruz D., et al. “Preparation and physicochemical characterization of Ag nanoparticles biosynthesized by Lippia citriodora (Lemon Verbena.” Colloids and Surfaces B: Biointerfaces 81.1(2010): 67-73.
- Aromal SA and Philip D. “Green synthesis of gold nanoparticles using Trigonella foenum-graecum and its size dependent catalytic activity”. Spectrochimica Acta Part A: Molecular and Biomolecular Spectroscopy 97 (2012): 1-5.
- Kim JS., et al. “Antimicrobial effects of silver nanoparticles”. Nanomedicine: Nanotechnology, Biology and Medicine 3.1(2007): 95-101.
- Jiang J., et al. “Characterization of size, surface charge, and agglomeration state of nanoparticle dispersions for toxicological studies”. Journal of Nanoparticle Research 11.1 (2009): 77-89.
- Feldheim DL Foss CA. M., et al. nanoparticles: synthesis, characterization, and applications. Boca Raton, FL: CRC Press; (2002).
- Sepeur S. Nanotechnology: technical basics and applications. Hannover: Vincentz; (2008).
- Shahverdi AR., et al. “Basic and practical procedures for microbial synthesis of nanoparticles. In: Rai M, Duran N, editors. Metal nanoparticles in microbiology”. Berlin: Springer; (2011): 177-197.
- Pal S., et al. “Does the antibacterial activity of silver nanoparticles depend on the shape of the nanoparticle? A study of the Gram-negative bacterium Escherichia coli”. Applied and Environmental Microbiology 73.6 (2007): (2007): 1712-1720.
- Huang H and Yang X. “Synthesis of polysaccharide-stabilized gold and silver nanoparticles: a green method”. Carbohydrate Research 339.15 (2004): 2627-2231.
- Shankar SS., et al. “Rapid synthesis of Au, Ag, and bimetallic Au core–Ag shell nanoparticles using Neem (Azadirachta indica) leaf broth”. Journal of Colloid and Interface Science 275.2 (2004): 496-502.
- Cao G. Nanostructures and nanomaterials: synthesis, properties and applications. London: Imperial College Press; (2004).
- Schaffer B., et al. “High-resolution surface plasmon imaging of gold nanoparticles by energy-filtered transmission electron microscopy”. Physical Review B 79 (2009).
- Eppler AS., et al. “Thermal and chemical stability and adhesion strength of Pt nanoparticle arrays supported on silica studied by transmission electron microscopy and atomic force microscopy”. The Journal of Physical Chemistry B - ACS Publications 104 (2000): 7286-7292.
- Chithrani BD., et al. “Determining the size and shape dependence of gold nanoparticle uptake into mammalian cells”. Nano Letters 6 (2006): 662-668.
- Sun S., et al. “Monodisperse FePt Nanoparticles and Ferromagnetic FePt Nanocrystal Superlattices”. Science 287.5460 (2000): 1989-1992.
- Strasser P., et al. “Lattice-strain control of the activity in dealloyed core–shell fuel cell catalysts”. Nature Chemistry 2 (2010): 454-460.
- Khatoon S and Ahmad T. “Synthesis, optical and magnetic properties of Ni-doped ZnO nanoparticles.” Materials Science and Engineering: B - Journal2012, 6, 325-333.
- Jayalakshmi M., et al. “Effect of particle size on the electrochemical capacitance of a-Ni(OH)2 in alkali solutions.” International Journal of Electrochemical Science 1 (2006): 324-333.
- Wijnhoven SWP., et al. “Nano-silver – a review of available data and knowledge gaps in human and environmental risk assessment”. Nanotoxicology 3 (2009): 109-138.
- Nel A., et al. “Toxic potential of materials at the nanolevel”. Science 311.5761 (2006): 622-627.
- Skebo JE., et al. “Assessment of metal nanoparticle agglomeration, uptake and interaction using high-illuminating system”. International Journal of Toxicology 26.2 (2007): 135-141.
- Wallace WE., et al. “Phospholipid lung surfactant and nanoparticle surface toxicity: lessons from diesel soots and silicate dusts”. Journal of Nanoparticle Research 9.1 (2007): 23-38.
- Bharali D J., et al. “Cross-linked polyvinylpyrrolidone nanoparticles: a potential carrier for hydrophilic drugs”. Journal of Colloid and Interface Science 258.2 (2003): 415-423.
- Mehata MS. “Stark shifts and exciton dissociation in CdSe nanoparticle grafted conjugated polymer”. Applied Physics Letters 100.15 (2012): 151908.
- Mehata MS. “Enhancement of charge transfer and quenching of photoluminescence of capped CdS quantum dots”. Scientific Reports 5 (2015).
- Mehata MS., et al. “External electric field effects on optical property and excitation dynamics of capped CdS quantum dots embedded in a polymer film”. Journal of Physical Chemistry 114.37 (2010): 15594-15601.
- Ratnesh RK and Mehata MS. “Controlled synthesis and optical properties of tunable CdSe quantum dots and effect of pH”. AIP Advances 5 (2015): 097114.
- Saxena A., et al. “Ultra-low sized cross-linked polyvinyl pyrrolidone nanoparticles as non-viral vectors for in vivo gene delivery”. Biomaterials 27.32 (2006): 5596-5602.
- Subbiah R., et al. “Nanoparticles: functionalization and multifunctional applications in biomedical sciences”. Current Medicinal Chemistry 17.36 (2010): 4559-4577.
- Malik P., et al. Green chemistry based benign routes for nanoparticle synthesis. Journal of Nanoparticles (2014).
- WeinerS and Dove PM. “An overview of biomineralization processes and the problem of the vital effect”. Reviews in Mineralogy and Geochemistry 54.1 (2003): 1-29.
- Bansal V., et al. “Inorganic materials using ‘unusual’microorganisms”. Advances in Colloid and Interface Science 179.182 (2012): 150-168.
- Dickson DP. “Nanostructured magnetism in living systems”. Journal of Magnetism and Magnetic Materials 203.1 (1999): 46-49.
- Lovley DR., et al. “Anaerobic production of magnetite by a dissimilatory ironreducing microorganism”. Nature 330.6145 (1987): 252-254.
- Philipse AP and Maas D. “Magnetic colloids from magnetotactic bacteria: chain formation and colloidal stability”. Langmuir 18.25 (2002): 9977-9984.
- Kroger N., et al. “Polycationic peptides from diatom biosilica that direct silica nanosphere formation”. Science 286.5442 (1999): 1129-1132.
- Mann S. “Molecular tectonics in biomineralization and biomimetic materials chemistry”. Nature 365.6446 (1993): 499-505.
- Oliver S., et al. “Lamellar aluminophosphates with surface patterns that mimic diatom and radiolarian microskeletons”. Nature (1995): 378.6552 47-50.
- Narayanan KB., et al. “Biological synthesis of metal nanoparticles by microbes”. Advances in Colloid and Interface Science 156.1 (2010): 1-13.
- Jha AK., et al. “Plant system: nature's nanofactory”. Colloids and Surfaces B: Biointerfaces 73.2 (2009): 219-223.
- Makarov V V., et al. “Green” nanotechnologies: synthesis of metal nanoparticles using plants”. Acta Naturae 6.1 (2014): 35.
- Mittal AK., et al. “Synthesis of metallic nanoparticles using plant extracts”. Biotechnology Advances 31.2 (2013): 346-356.
- Sohn JS., et al. DNA-templated preparation of gold nanoparticles. Molecules 16.10 (2011): 8143-8151.
- Ahmad A., et al. “Enzyme mediated extracellular synthesis of CdS nanoparticles by the fungus, Fusarium oxysporum”. Journal of the American Chemical Society 124. 41 (2002): 12108-12109.
- Kasra Momeni., et al. “Structural transformation in monolayer materials: a 2D to 1D transformation”. Physical Chemistry Chemical Physics 18 (2016): 19873-19879.
- Joerger R., et al. “Biologically produced silver–carbon composite materials for optically functional thin-film coatings”. Advanced Materials 12.6 (2000): 407–409.
- Panigrahi S., et al. “General method of synthesis for metal nanoparticles”. Journal of Nanoparticle Research 6.4 (2004): 411–414.
- Oliveira MM., et al. “Influence of synthetic parameters on the size, structure, and stability of dodecanethiol-stabilized silver nanoparticles”. Journal of Colloid and Interface Science 292.2 (2005): 429–435.
- Gan PP., et al. “Green synthesis of gold nanoparticles using palm oil mill effluent (POME): a low-cost and eco-friendly viable approach”. Bioresource Technology 113 (2012): 132–135.
- Sathish kumar M., et al. “Cinnamon zeylanicum bark extract and powder mediated green synthesis of nano-crystalline silver particle and its bactericidal activity”. Colloids and Surfaces B: Biointerfaces 73.2 (2009): 332–338.
- Iravani S. “Critical review – green synthesis of metal nanoparticles using plants”. Green Chemistry 13 (2011): 2638–2650.
- Shivaji S., et al. “Extracellular synthesis of antibacterial silver nanoparticles using psychrophilic bacteria”. Process Biochemistry 46.9 (2011): 1800–1807.
- Stephen JR and Macnaughtont SJ. “Developments in terrestrial bacterial remediation of metals”. Current Opinion in Biotechnology 10.3 (1999): 230–233.
- Chan YS and Mat Don M. “Biosynthesis and structural characterization of Ag nanoparticles from white rot fungi”. Materials Science and Engineering: C 33.1 (2013): 282–288.
- Syed A., et al. “Biological synthesis of silver nanoparticles using the fungus Humicola sp. And evaluation of their cytoxicity using normal and cancer cell lines”. Spectrochimica Acta Part A: Molecular and
- Biomolecular Spectroscopy 114 (2013): 144–147.
- Kumar KS., et al. “Synthesis of gold nanoparticles: an ecofriendly approach using Hansenula anomala”. ACS Applied Materials & Interfaces 3.5 (2011): 1418–1425.
- Akhtar MS., et al. “Biogenic synthesis of metallic nanoparticles by plant extracts”. ACS Sustainable Chemistry & Engineering 1.6 (2013): 591–602.
- Kanchi S., et al. “Exploitation of de-oiled jatropha waste for gold nanoparticles synthesis: a green approach”. Arabian Journal of Chemistry 10 (2014): 10.
- Hakim LF., et al. “Aggregation behavior of nanoparticles in fluidized beds”. Powder Technology 160.3 (2005): 149–160.
- Mukherjee P., et al. “Fungus-mediated synthesis of silver nanoparticles and their immobilization in the mycelial matrix: a novel biological approach to nanoparticle synthesis”. Nano Letter 1.10 (2001): 515–519.
- Tripp SL., et al. “Self-assembly of cobalt nanoparticle rings”. Journal of the American Chemical Society 124.27 (2002): 7914–7915.
- Huang J., et al. “Bio inspired synthesis of metal nanomaterial’s and applications. Chemical Society Reviews 44.17 (2015): 6330–6374.
- Riddin T., et al. “Biological synthesis of platinum nanoparticles: effect of initial metal concentration”. Enzyme and Microbial Technology 46.6 (2010): 501–505.
- Adam Schröfel., et al. “Applications of biosynthesized metallic nanoparticles- a review”. Acta Biomaterialia 10.10 (2014): 4023- 4042.
- Mishra A., et al. “Fungus mediated synthesis of gold nanoparticles and their conjugation with genomic DNA isolated from Escherichia coli and Staphylococcus aureus”. Process Biochemistry 47. 5 (2012): 701-711.
- Yallappa, S., et al. “Phytosynthesis of stable Au, Ag and Au–Ag alloy nanoparticles using J. sambac leaves extract, and their enhanced antimicrobial activity in presence of organic antimicrobials”. Spectrochimica Acta Part A: Molecular and Biomolecular Spectroscopy 137 (2015): 236–243.
- Khalil MMH., et al. “Biosynthesis of Au nanoparticles using olive leaf extract: 1st Nano Updates”. Arabian Journal of Chemistry 5.2 (2012): 431–437.
- Wang Z., et al. “Characterization of iron–polyphenol nanoparticles synthesized by three plant extracts and their fenton oxidation of azo dye”. ACS Sustainable Chemistry & Engineering 2.4 (2014): 1022–1025.
- Patel V., et al. “Screening of cyanobacteria and microalgae for their ability to synthesize silver nanoparticles with antibacterial activity”. Biotechnology Reports 5 (2015): 112–119.
- Sepeur S. “Nanotechnology: technical basics and applications”. Hannover: Vincentz (2008):
- Meyers MA ., et al. “Mechanical properties of nanocrystalline materials”. Progress in Materials Science 51.4 (2006): 427–556.
- Thakkar KN., et al. “Biological synthesis of metallic nanoparticles”. Nanomedicine: Nanotechnology, Biology and Medicine 6.2 (2010): 257-262.
- Dhillon GS., et al. “Green approach for nanoparticle biosynthesis by fungi: current trends and applications”. Critical Reviews in Biotechnology 32.1 (2012): 49–73.
- Gericke M and Pinches A. “Biological synthesis of metal nanoparticles”. Hydrometallurgy 83.1-4 (2006): 132-140.
- Kaler A., et al. “Extracellular biosynthesis of silver nano- particles using aqueous extract of Candida viswanathii”. Journal of Bionanoscience 5.1 (2011): 53-58.
- Korbekandi H., et al. “Production of nanoparticles using organisms”. Critical Reviews in Biotechnology 29.4 (2009): 279–306.
- Li X., et al. “Biosynthesis of nanoparticles by microorganisms and their applications”. Journal of Nanomaterials 2011(2011): 16.
- Luangpipat T., et al. “Gold nanoparticles produced in a microalga”. Journal of Nanoparticle Research 13.12 (2011): 6439–6345.
- Mohanpuria P., et al. “Biosynthesis of nanoparticles: technological concepts and future applications”. Journal of Nanoparticle Research 10.3 (2008): 507-517.
- Sanghi R and Verma P. “Microbes as green and eco-friendly nanofactories”. Green Chemistry for Environmental Sustainability 15 (2010): 315-339.
- Sastry M., et al. “Biosynthesis of metal nanoparticles using fungi and actinomycete”. Current Science 85.2 (2003): 162-170.
- Gardea-Torresdey JL., et al. “Alfalfa sprouts: a natural source for the synthesis of silver nanoparticles”. Langmuir 19.4 (2003): 1357-1361.
- Park Y., et al. “Polysaccharides and phytochemicals: a natural reservoir for the green synthesis of gold and silver nanoparticles”. IET Nanobiotechnology 5.3 (2011): 69–78.
- Dubey M., et al. “Green synthesis of nanosilver particles from extract of Eucalyptus hybrida (safeda) leaf”. Digest Journal of Nanomaterials and Biostructures 4.3 (2009): 537-543.
- Huang JL., et al. “Biosynthesis of silver and gold Nanoparticles by novel sundried Cinnamomum camphora leaf”. Nanotechnology (2007): 18.
- Tripathy, A., et al. “Process variables in biomimetic synthesis of silver nanoparticles by aqueous extract of Azadirachta indica (Neem) leaves”. Journal of Nanoparticle Research 12 (2010): 237–246.
- Ramanathan R., et al. “Aqueous phase synthesis of copper nanoparticles: a link between heavy metal resistance and nano- particle synthesis ability in bacterial systems”. Nanoscale 21 (2013): 2300-2306.
- Sangeetha G., et al. “Green synthesis of zinc oxide nanoparticles by aloe barbadensis miller leaf extract: structure and optical properties”. Materials Research Bulletin 46.12 (2011): 2560–2566.
- Jha AK., et al. “Biosynthesis of silver nanoparticles using Eclipta leaf”. Biotechnology Progress 25 (2009): 1475–1477.
- Dwivedi AD and Gopal K. “Biosynthesis of silver and gold nanoparticles using Chenopodium album leaf extract”. Colloids and Surfaces A: Physicochemical and Engineering Aspects 369.1-3(2010): 27–33.
- Chandran SP., et al. “Synthesis of gold nanotriangles and silver nanoparticles using Aloe vera plant extract”. Biotechnology Progress 22.2 (2006): 577-583.
- Dubey SP., et al. “Green synthesis and characterizations of silver and
- gold nanoparticles using leaf extract of Rosa rugosa”. Colloids and Surfaces A: Physicochemical and Engineering Aspects 364.1-3 (2010): 34–41
- Dubey SP., et al. “Tansy fruit mediated greener synthesis of silver and gold nanoparticles”. Process Biochemistry 45.7 (2010): 1065-1071.
- Palaniselvam Kuppusamy., et al. “Biosynthesis of metallic nanoparticles using plant derivatives and their new avenues in pharmacological applications – An updated report”. Saudi Pharmaceutical Journal 24.4 (2016): 473-484.
- Noruzi M., et al.“Rapid green synthesis of gold nanoparticles using Rosa hybrida petal extract at room temperature”. Spectrachem. Acta Part A79.5 (2011): 1461-1465.
- Das RK., et al.“Green synthesis of gold nanoparticles using Nyctanthes arbortristis flower extract”. Bioprocess and Biosystems Engineering 34.5 (2011): 615-619.
- Vankar PS and Bajpai D. “Preparation of gold nanoparticles from Mirabilis jalapa flowers”. Indian Journal of Biochemistry & Biophysics 47.3(2010): 157-160.
- Gardea-Torresdey JL., et al. “Gold nanoparticles obtained by bio-precipitation from gold (III) solutions”. Journal of Nanoparticle Research 1.3 (1999): 397-404.
- Huang J., et al. “Biosynthesis of silver and gold nanoparticles by novel sundried Cinnamomum camphora leaf”. Nanotechnology 18.10 (2007): 1-11.
- Ahmad N and Sharma S. “Green synthesis of silver nanoparticles using extracts of Ananas comosus”. Green and Sustainable Chemistry 2.4 (2012): 141-147.
- Sathishkumar M., et al. “Immobilization of silver nanoparticles synthesized using the Curcuma longa tuber powder extract on cotton cloth for bactericidal activity”. Bioresource Technology 101.20 (2010): 7958-7965.
- Song JY., et al. “Biological synthesis of gold nanoparticles using Magnolia kobus and Diopyros kaki leaf extracts”. Process Biochemistry 44.10 (2009): 113-138.
- Kiranmai Mandava. “Biological and Non-biological Synthesis of Metallic Nanoparticles: Scope for Current Pharmaceutical Research”. Indian Journal of Pharmaceutical Sciences 79.4 (2017): 501-512.
- Shankar SS., et al. “Geranium leaf assisted biosynthesis of silver nanoparticles”. Biotechnology Progress 19.6 (2003): 1627-1631.
- Raju D., et al.“Synthesis of gold nanoparticles by various leaf fractions of Semecarpus anacardium L”. Trees 25.2 (2011): 145-151.
- Marchiol L., et al. “Synthesis of metal nanoparticles in living plants”. Italian Journal of Agronomy 7.3 (2012): 37-44.
- Rai AA., et al.“Role of halide ions and temperature on the morphology of biologically synthesized gold nanotriangles”. Langmuir 22.2 (2006): 736-741.
- Gittins DI., et al.“A nanometre-scale electronic switch consisting of a metal cluster and redox-addressable groups”. Nature 408.6808 (2000): 67-69.
- Liu FK., et al. “Studying the size/shape separation and optical properties of silver nanoparticles by capillary electrophoresis”. Journal of Chromatography A 1062.1 (2005): 139-145.
- Maruyama T., et al.“Synthesis of gold nanoparticles using various amino acids”. Journal of Colloid and Interface Science 447 (2015): 254-257.
- Polavarapu L and Xu QH. “A single-step synthesis of gold nanochains using an amino acid as a capping agent and characterization of their optical properties”. Nanotechnology 19.7 (2008): 075601.
- Xu S., et al. “Zwitterion formation in hydrated amino acid, dipole bound anions: how many water molecules are required?” The Journal of Chemical Physics 119.20 (2003): 10696-10701.
- Akhlaghi SP., et al. “Sustainable nanomaterials derived from polysaccharides and amphiphilic compounds”. Soft Matter 9.33 (2013): 7905-7918.
- Duan H., et al. “Green chemistry for nanoparticle synthesis”. Chemical Society Reviews 44.16 (2015): 5778-5792.
- Boury B and Plumejeau S. “Metal oxides and polysaccharides: an efficient hybrid association for materials chemistry”. Green Chemistry 17.1 (2015): 72-88.
- Bao SJ., et al.“Environmentally-friendly biomimicking synthesis of TiO2 nanomaterials using saccharides to tailor morphology, crystal phase and photocatalytic activity”. CrystEngComm15.23 (2013): 4694-4699.
- Virkutyte J andVarma RS. “Green synthesis of metal nanoparticles:biodegradable polymers and enzymes in stabilization and surface functionalization”. Chemical Science 2.5 (2011): 837-846.
- Philip D., et al. “Biosynthesis of Au, Ag and Au–Ag nanoparticles using edible mushroom extract”. Spectrochimica Acta Part A: Molecular and Biomolecular Spectroscopy 73.2 (2009): 374-381.
- Cheng F., et al. “Synthesis and antibacterial effects of aqueous colloidal solutions of silver nanoparticles using aminocellulose as a combined reducing and capping reagent”. Green Chemistry 15.4 (2013): 989-998.
- Feng J., et al. “Adsorption mechanism of single amino acid and surfactant molecules to Au 111 surfaces in aqueous solution: design rules for metal-binding molecules”. Soft Matter 7.5 (2011): 2113-2120.
- Jo JH., et al. “Pseudomonas deceptionensis DC5-medi-ated synthesis of extracellular silver nanoparticles”. Artificial Cells, Nanomedicine, and Biotechnology 44.6 (2015): 1576-1581.
- Singh P., et al. “Weissella oryzae DC6-facilitated green synthesis of silver nanoparticles and their antimicrobial potential”. Artificial Cells, Nanomedicine, and Biotechnology 44.6 (2015): 1569-1575.
- Wang C., et al. “Green synthesis of silver nanoparticles by Bacillus methylotrophicus, and their antimicrobial activity”. Artificial Cells, Nanomedicine, and Biotechnology 44.4 (2015): 1127-1132.
- Singh P., et al. “Biosynthesis, characterization, and antimi-crobial applications of silver nanoparticles”. International Journal of Nanomedicine 10 (2015): 2567-2577.
- Singh P., et al. “Biosynthesis of anisotropic silver nano-particles by Bhargavaea indica and their synergistic effect with antibiotics against pathogenic microorganisms”.Journal of Nanomaterials 2015.234741 (2015): 10.
- Singh P., et al. “Microbial synthesis of flower-shaped gold nanoparticles”. Artificial Cells, Nanomedicine, and Biotechnology 44.6 (2015): 1469-1474.
- Singh BR., et al. “Synthesis of stable cadmium sulfide nanoparticles using surfactin produced by Bacillus amyloliquifa-ciens strain KSU-109”. Colloids and Surfaces B: Biointerfaces 85.2 (2011): 207-213.
- Bai H., et al. “Biological synthesis of size-controlled cad-mium sulfide nanoparticles using immobilized Rhodobacter sphaeroides”. Nanoscale Research Letters 4.7 (2009): 717-723.
- Elbeshehy EK., et al. “Silver nanoparticles synthesis medi-ated by new isolates of Bacillus spp., nanoparticle characterization and their activity against bean yellow mosaic virus and human pathogens”. Frontiers in Microbiology6 (2015): 453.
- Soni N., et al. “Antimicrobial and mosquitocidal activity of microbial synthesised silver nanoparticles”. Parasitology Research 114 (2015): 1023-1030.
- Zhang X., et al. “Synthesis of nanoparticles by microorgan-isms and their application in enhancing microbiological reaction rates”. Chemosphere 82.1 (2011): 489-494.
- Alghuthaymi MA., et al. “Myconanoparticles: synthesis and their role in phytopathogens management”. Biotechnology & Biotechnological Equipment 29.2 (2015): 221-236.
- Castro-Longoria E., et al. “Biosynthesis of silver, gold and bimetallic nanoparticles using the filamentous fungus Neurospora crassa”. Colloids and Surfaces B: Biointerfaces 83.1 (2011): 42-48.
- Anil Kumar S., et al. “Nitrate reductase-mediated synthesis of silver nanoparticles from AgNO3”. Biotechnology Letters 29 (2007): 439-445.
- Golinska P., et al. “Biogenic synthesis of metal nanoparticles from actinomycetes: biomedical applications and cytotoxicity”. Applied Microbiology and Biotechnology 98.19 (2014): 8083-8097.
- Crookes-Goodson WJ., et al. “Bio-directed synthesis and assembly of nanomaterials”. Chemical Society Reviews 37.11 (2008): 2403-2412.
- Gade AK., et al. “Exploitation of Aspergillus niger for synthesis of silver nanoparticles”. Journal of Biobased Materials and Bioenergy 2.3 (2008): 243-247.
- Karthik L., et al. “Streptomyces sp. LK3 mediated synthesis of silver nanoparticles and its biomedical application”. Bioprocess and Biosystems Engineering 37.2 (2014): 261-267.
- Apte M., et al. “Psychrotrophic yeast Yarrowia lipolytica NCYC 789 mediates the synthesis of antimicrobial silver nano-particles via cell-associated melanin”. AMB Express 3 (2013): 32.
- Seshadri S., et al. “Green synthesis of lead sulfide nano-particles by the lead resistant marine yeast, Rhodosporidium diobovatum”. Biotechnology Progress 27.5 (2011): 1464-1469.
- Mourato A., et al. “Biosynthesis of crystalline silver and gold nanoparticles by extremophilic yeasts”. Bioinorganic Chemistry and Applications 2011 (2011): 546074.
- Waghmare SR., et al. “Ecofriendly production of silver nanoparticles using Candida utilis and its mechanistic action against pathogenic microorganisms”.3 Biotech 5.1 (2015): 33-38.
- Chiu CY., et al “Biomolecular specificity controlled nanomaterial synthesis”. Chemical Society Reviews 42.7(2013):2512-2527.
Xia Y., et al. “One-dimensional nanostructures: synthesis, characterization, and applications”. Advanced Materials 15.5 (2003): 353-389. - Singaravelu G., et al. “A novel extracellular synthesis of monodisperse gold nanoparticles using marine alga, Sargassum wightii Greville”. Colloids and Surfaces B: Biointerfaces 57.1 (2007): 97-101.
- Arockiya Aarthi Rajathi F., et al. “Biosynthesis of antibacterial gold nanoparticles using brown alga, Stoechospermum marginatum (kutzing)”. Spectrochimica Acta Part A: Molecular and Biomolecular Spectroscopy 99 (2012): 166-173.
- Sicard C., et al. “Nano-gold biosynthesis by silica-encapsulated Micro-algae: a ‘‘living” bio-hybrid material”. Journal of Materials Chemistry 20.42 (2010): 9342-9347.
- Ghosh, P., et al. “Gold nanoparticles in delivery applications”. Advanced Drug Delivery Reviews 60.11 (2008): 1307-1315.
- Hu MS., et al. “Photosensitive gold-nanoparticle embedded dielectric nanowires”. Nature Materials 5.2 (2006): 102-106.
- Mikami Y., et al. “Catalytic activity of unsupported gold nanoparticles”. Catalysis Science & Technology 3.1 (2013): 58-69.
- Nair B and Pradeep T. “Coalescence of nanoclusters and formation of submicron crystallites assisted by Lactobacillus strains”. Crystal Growth & Design 2.4 (2002): 293-298.
- Pingarro n JM., et al. “Gold nanoparticle-based electrochemical biosensors”. Electrochimica Acta 53.19 (2008): 5848-5866.
- Zhao Y., et al. “Small molecule-capped gold nanoparticles as potent antibacterial agents that target gram-negative bacteria”. Journal of the American Chemical Society 132.35 (2010): 12349-12356.
- Sharma B., et al. “Biosynthesis of fluorescent gold nanoparticles using an edible freshwater red alga,
- Lemanea fluviatilis (L.) C.Ag. and antioxidant activity of biomatrix loaded nanoparticles”. Bioprocess and Biosystems Engineering 37.12 (2014): 2559-2565.
- Sharma B., et al. “Biosynthesis of gold nanoparticles using a freshwater green alga, Prasiola crispa”. Materials Letters 116 (2014): 94-97.
- Parial D and Pal R. “Biosynthesis of monodisperse gold nanoparticles by green alga Rhizoclonium and associated biochemical changes”. Journal of Applied Phycology 27.2 (2015): 975-984.
- Li X., et al. “Biosynthesis of nanoparticles by microorganisms and their applications”. Journal of Nanomaterial’s (2011).
- Dadosh T. “Synthesis of uniform silver nanoparticles with a controllable size”. Materials Letters 63.1.26 (2009): 2236-2238.
- Shakeel A., et al. “A review on plants extract mediated synthesis of silver nanoparticles for antimicrobial applications: A green expertise”. Journal of Advanced Research 7.1 (2015): 17-28.
- Palaniyandi Velusamy., et al. “Bio-Inspired Green Nanoparticles: Synthesis, Mechanism, and Antibacterial Application”. Toxicology Research 32.2 (2016): 95-102.
- Zhai T., et al. “A Comprehensive review of one-dimensional metal-oxide nanostructure photodetectors”. Sensors 9.8 (2009): 6504-6529.
- Gotic M and Music S. “Synthesis of nanocrystalline iron oxide particles in the iron (III) acetate/alcohol/acetic acid system”. European Journal of Inorganic Chemistry 6 (2008): 966-973.
- Hayashi H and Hakuta Y 2010. “Hydrothermal synthesis of metal oxide nanoparticles in supercritical water”. Materials 3.7 (2010): 3794-3817.
- Kim CS., et al. “Solvothermal synthesis of nanocrystalline TiO2 in toluene with surfactant”. Journal of Crystal Growth 257.3.4 (2003): 309-315.
- Leonelli C and Lojkowski W. “Main development directions in the application of microwave irradiation to the synthesis of nanopowders”. Chimica oggi 25 (2007): 34-38.
- Abboud Y., et al. “Biosynthesis, characterization and antimicrobial activity of copper oxide nanoparticles (CONPs) Produced using brown alga extract (Bifurcaria bifurcata)”. Applied Nanoscience 4.5 (2014): 571-576.
- Nagarajan S., et al. “Extracellular synthesis of zinc oxide nanoparticle using seaweeds of Gulf of Mannar, India”. Journal of Nanobiotechnology 11.1 (2013): 11-39.
- Mahdavi M., et al. “Green biosynthesis and characterization of magnetic iron oxide (Fe3O4) nanoparticles using seaweed (Sargassum muticum) aqueous extract”. Molecules 18.5 (2013): 5954-5964.
- Miyashita K., 2009. “The carotenoid fucoxanthin from brown seaweed affects obesity”. Lipid Technology 21.8.9 (2009): 186-190.
- Namvar F., et al. “Polyphenol-rich seaweed (Eucheuma cottonii) extract suppresses breast tumour via hormone modulation and apoptosis induction”. Food Chemistry 130.2 (2012): 376-382.
- Nishino T., et al. “Inhibition of the generation of thrombin and factor Xa by a fucoidan from the brown seaweed Ecklonia kurome”. Thrombosis Research 96.1 (1999): 37-49.
- Perez GR., et al. “Antidiabetic effect of compounds isolated from plants”. Phytomedicine 5.1 (1998): 55-75.
- Azizi S., et al. “Green biosynthesis and characterization of zinc oxide nanoparticles using brown marine macroalga Sargassum muticum aqueous extract”. Materials Letters 116 (2014): 275-277.
- Francavilla M., et al. “Efficient and simple reactive milling preparation of photocatalytically active porous ZnO nanostructures using biomass derived polysaccharides”. Green Chemistry 16.5 (2014): 2876-2885.
- Bianchi CL., et al. “Nanocrystalline oxides: surfactants-assisted growth”. Dekker Encyclopedia of Nanoscience and Nanotechnology (2006): 1-10
- Liu L., et al. “Are microorganisms indispensable in green microbial nanomaterial synthesis?” RSC Advances 4.28 (2014): 14564-14568.
- Alanazi FK., et al. “Biopharmaceutical applications of nanogold”. Saudi Pharmaceutical Journal 18.4 (2010): 179-193.
- Singh S., et al. “A novel ‘green’ synthesis of colloidal silver nanoparticles (SNP) using Dillenia indica fruit extract”. Colloids and Surfaces B: Biointerfaces 102 (2013): 83-85.
- Narayanan KB and Sakthivel N. “Green synthesis of biogenic metal nanoparticles by terrestrial and aquatic phototrophic and heterotrophic eukaryotes and biocompatible agents”. Advances in Colloid and Interface Science 169.2 (2011): 59-79.
- Sylvie Gaillet and Jean-Max Rouanet. “Silver nanoparticles: Their potential toxic effects after oral exposure and underlying mechanisms –A review”. Food and Chemical Toxicology 77 (2015): 58-63.
- Jie J., et al. “One-dimensional II–VI nanostructures: Synthesis, properties and optoelectronic applications”. Nano Today 5.4 (2010): 313-336.
- Communication from the Commission to the European Parliament, the Council and the European Economic and Social Committee, The second regulatory review dedicated to Nanomaterials, Brussels, 2012.
- Kikuchi M. “Nanotechnology impact towards biomedical engineering”. Biocybernetics and Biomedical Engineering 27 (2007): 27-32.
- Cheng MM., et al. “Nanotechnologies for biomolecular detection and medical diagnostics”. Current Opinion in Chemical Biology 10.1 (2006): 11-19.
- Logothetidis S., “Nanotechnology in medicine: The medicine of tomorrow and nanomedicine”. Hippokratia 10.1 (2006): 7-21.
- Morganti P. “Use and potential of nanotechnology in cosmetic dermatology”. Clinical, Cosmetic and Investigational Dermatology 3 (2010): 5-13.
- Sharma A., et al. “Nanotechnology: a promising approach for cosmetics”. International Journal of Pharmaceutical Sciences and Research 2 (2012): 54-61.
- Darbre PD., et al. “Concentrations of parabens in human breast tumours”. Journal of Applied Toxicology 24.1 (2004): 5-13.
- Sainio EL., et al. “Ingredients and safety of cellulite creams”. European Journal of Dermatology 10.8 (2000): 596-603.
- Cross SE and Roberts MS. “The effect of occlusion on epidermal penetration of parabens from a commercial allergy test ointment, acetone and ethanol vehicles”. Journal of Investigative Dermatology 115.5 (2000): 914-918.
- Handa O., et al. “Methylparaben potentiates UV-induced damage of skin keratinocytes”. Toxicology 227.1.2 (2006): 62-72.
- Ishiwatari S., et al. “Effects of methyl paraben on skin keratinocytes”. Journal of Applied Toxicology 27.1 (2007): 1-9.
- Kokura S., et al. “Silver nanoparticles as a safe preservative for use in cosmetics”. Nanomedicine: Nanotechnology 6.4 (2010): 570-574.
- Davies JC. “Oversight of next generation nanotechnology”. Woodrow Wilson International Center for Scholars, Project on Emerging Nanotechnologies (2009).
- Kouvaris P., et al. “Green synthesis and characterization of silver nanoparticles produced using Arbutus unedo leaf extract”. Materials Letters 76 (2012): 18-20.
- Kumar V and Yadav SK. “Plant-mediated synthesis of silver and gold nanoparticles and their applications”. Journal of Chemical Technology and Biotechnology 84.2 (2009): 151-157.
- Petla RK., et al. “Soybean (Glycine Max) leaf extract based green synthesis of palladium nanoparticles”. Journal of Biomaterials and Nanobiotechnology 3.1 (2012): 14-19.
- Magnacca G., et al. “Novel magnetite nanoparticles coated with waste-source biobased substances as sustainable and renewable adsorbing materials”. ACS Sustainable Chemistry & Engineering 2.6 (2014): 1518-1524.
- Eroglu E., et al. “Biogenic production of palladium nanocrystals using microalgae and their immobilization on chitosan nanofibers for catalytic applications”. RSC Advances 3.4 (2013): 1009-1012.
- Parker HL., et al. “Direct synthesis of Pd nanoparticles on alginic acid and seaweed supports”. Green Chemistry 17.4 (2015): 2200-2207.
- Kuppusamy P., et al. “Biosynthesized gold nanoparticle developed as a tool for detection of HCG hormone in pregnant women urine sample”. Asian Pacific Journal of Tropical Disease 4.3 (2014): 237.
- Vlastimil Peksa., et al. “Testing gold nanostructures fabricated by hole-mask colloidal lithography as potential substrates for SERS sensors: sensitivity, signal variability, and the aspect of adsorbate deposition”. Physical Chemistry Chemical PhysicsPhys 18.29 (2016): 19613-19620.
- Anu Tresa Sunny., et al. “Copper oxide nanoparticles in an epoxy network: microstructure, chain confinement and mechanical behaviour”. Physical Chemistry Chemical Physics 18.29 (2016): 19696-19704.
- Brondani D., et al. “Biosensor based on platinum nanoparticles dispersed in ionic liquid and laccase for determination of adrenaline”. Sensors and Actuators B: Chemical 140.1 (2009): 252-259.
- Zhang Y., et al. “Chloroplasts-mediated biosynthesis of nanoscale Au–Ag alloy for 2-butanone assay based on electrochemical sensor”. Nanoscale Research Letters 7.1 (2012): 475.
- Myeongjin Kim., et al. “Design and synthesis of ternary Co3O4/carbon coated TiO2 hybrid nanocomposites for asymmetric supercapacitors”. Physical Chemistry Chemical Physics 18.29 (2016): 19696-19704.
- Xiaoxiao Liu., et al. “Direct planting of ultrafine MoO2+δ nanoparticles in carbon nanofibers by electrospinning: self-supported mats as binder-free and long-life anodes for lithium-ion batteries”. Physical Chemistry Chemical Physics 18.29 (2016): 19832-19837.
- Songand JY and Kim BS. “Biological synthesis of metal nanoparticles”. Biocatalysis and Agricultural Biotechnology (2009): 399-407.
- Weiss J., et al. “Functional materials in food nanotechnology”. Journal of Food Science 71.9 (2006): 107-116.
- Catherine CB and Adam SGC. “Functionalisation of magnetic nanoparticles for applications in biomedicine”. Journal of Physics D: Applied Physics 36.13 (2003): 36-198.
- Pankhurst QA., et al. “Applications of magnetic nanoparticles in biomedicine”. Journal of Physics D: Applied Physics 36.13 (2003): R167.
- Gupta AK and Gupta M. “Synthesis and surface engineering of iron oxide nanoparticles for biomedical applications”. Biomaterials 26.18 (2005): 3995-4021.
- Khan MS., et al. “Gold nanoparticles: a paradigm shift in biomedical applications”. Advances in Colloid and Interface Science 199.200 (2013): 44-58.
- Cai W., et al. “Applications of gold nanoparticles in cancer nanotechnology”. Nanotechnology, Science and Applications 1 (2008): 17-32.
- van Horssen R., et al. “TNFalpha in cancer treatment: molecular insights, antitumor effects, and clinical utility”. Oncologist 11.4 (2006): 397-408.
- Visaria RK., et al. “Enhancement of tumor thermal therapy using gold nanoparticle-assisted tumor necrosis Factor-alpha delivery”. Molecular Cancer Therapeutics 5.4 (2006): 1014-1020.
- Uma Suganya KS., et al. “Blue green alga mediated synthesis of gold nanoparticles and it antibacterial efficacy against gram positive organisms”. Materials Science and Engineering: C 47 (2015): 351-356.
- Nel AE., et al. “Understanding biophysicochemical interactions at the nano-bio interface”. Nature Materials 8.7 (2009): 543-557.
- Jain D., et al. “Synthesis of plant-mediated silver nanoparticles using papaya fruit extract and evaluation of their antimicrobial activities”. Digest Journal of Nanomaterials and Biostructures 4.3 (2009): 557-63.
- Krishnaraj C., et al. “Synthesis of silver nanoparticles using Acalypha indica leaf extracts and its antibacterial activity against water borne pathogens”. Colloids and Surfaces B: Biointerfaces 76.1 (2010): 50-56.
- Sondi I and Salopek-Sondi B. “Silver nanoparticles as antimicrobial agent:a case study on E. coli as a model for Gram-negative bacteria”. Journal of Colloid and Interface Science 275.1 (2004): 177-82.
- Kasthuri J., et al. “Phyllanthin assisted biosynthesis of silver and gold nanoparticles: a novel biological approach”. Journal of Nanoparticle Research 11.5 (2009): 1075-1085.
- Kim JS., et al. “Antimicrobial effects of silver nanoparticles”. Nanomedicine 3.1 (2007): 95-101.
- Ren G., et al. “Characterization of copper oxide nanoparticles for antimicrobial application”. International Journal of Antimicrobial Agents 33.6 (2009): 587-590.
- Ruparelia JP., et al. “Strain specificity in antimicrobial activity of silver and copper nanoparticles”. Acta Biomaterialia 4.3 (2008): 707-716.
- Kirsner R., et al. “Matrix metalloproteinase in normal and impaired wound healing: a potential role of monocrystalline silver”. Wounds 13 (2001): 5-10.
- Tian J., et al. “Tropical delivery of silver nanoparticles promotes wound healing”. Chem Med Chem 2.1 (2007): 129-136.
- Gurunathan S., et al. “Antiangiogenic properties of silver nanoparticles”. Biomaterials 30.31 (2009): 6341-6350.
- Vivek M., et al. “Biogenic silver nanoparticles by Gelidiella acerosa extract and their antifungal effects”. Avicenna Journal of Medical Biotechnology 3.3 (2011): 143-148.
- Logeswari P., et al “Synthesis of silver nanoparticles using plant extracts and analysis of their antimicrobial activity”. Journal of Saudi Chemical Society 4 (2012): 23-45.
- Rajakumar G and Abdul Rahuman A. “Larvicidal activity of synthesized silver nanoparticles using Eclipta prostrate leaf extract against filariasis and malaria vectors”. Acta Tropica 118.3 (2011): 196-203.
- Santhoshkumar T., et al. “Synthesis of silver nanoparticles using Nelumbo nucifera leaf extract and its larvicidal activity against malaria and filariasis vectors”. Parasitology Research 108.3 (2011): 693-702.
- Gnanadesigan M., et al. “Biosynthesis of silver nanoparticles by using mangrove plant extract and their potential mosquito larvicidal property”. Asian Pacific Journal of Tropical Medicine 4.10 (2011): 799-803.
- Jayaseelan C., et al. “Synthesis of pediculocidal and larvicidal silver nanoparticles by leaf extract from heart leaf moon seed plant Tinospora cordifolia Miers”. Parasitology Research 109.1 (2011):185-194.
- Raghunandan D., et al. “Anti-cancer studies of noble metal nanoparticles synthesized using different plant extracts”. Cancer Nanotechnology 2.1.6 (2011): 57-65.
- Das S., et al. “Biosynthesized silver nanoparticles by ethanolic extracts of Phytolacca decandra, Gelsemium sempervirens, Hydrastis canadensis and Thuja occidentalis induce differential cytotoxicity through G(2/M arrest in A375 cells”. Colloids and Surfaces B: Biointerfaces 101 (2013): 325-336.
- Dipankar C and Murugan S. “The green synthesis, characterization and evaluation of the biological activities of silver nanoparticles synthesized from Iresine herbstii leaf aqueous extracts”. Colloids and Surfaces B: Biointerfaces 98 (2012):112-119.
- Suriyakalaa U., et al. “Hepatocurative activity of biosynthesized silver nanoparticles fabricated using Andrographis paniculata”. Colloids and Surfaces B: Biointerfaces 102 (2013): 189-194.
- Swarnalatha C., et al. “Evaluation of in vitro antidiabetic activity of Sphaeranthus amaranthoides silver nanoparticles”. International Journal of Nanomaterials and Biostructures 2 (2012): 25-29.
- Pickup JC., et al. “Nanomedicine and its potential in diabetes research and practice”. Diabetes/Metabolism Research and Reviews 24.8 (2008): 604-610.
- Mary EJ and Inbathamizh L. “Green synthesis and characterization of nano silver using leaf extract of Morinda pubescens”. Asian Journal of Pharmaceutical and Clinical Research 5 (2012): 159-162.
- Reichelt KV., et al. “Phytochemical characterization of South African bush tea (Athrixia phylicoides DC)”. South African Journal of Botany 83 (2012): 1-8.
- Anil Kumar S and Khan MI. “Hetero functional nanomaterials: fabrication, properties and applications in nanobiotechnology”. Journal of Nanoscience and Nanotechnology 10.7 (2010): 4124-4134.
- Skirtach AG., et al. “Laser-induced release of encapsulated materials inside living cells”. Angewandte Chemie 45.28 (2006): 4612-4617.
- Sershen SR., et al. “Temperature-sensitive polymer-nanoshell composites for photothermally modulated drug delivery”. Journal of Biomedical Materials Research 51.3 (2000): 293.
- Gu H., et al. “Presenting vancomycin on nanoparticles to enhance antimicrobial activities”. Nano Letters 3.9 (2003): 1261-1263.
- Estella-Hermoso de Mendoza A., et al. “Lipid nanomedicines for anticancer drug therapy”. Journal of Biomedical Nanotechnology 5.4 (2009): 323-343.
- Saravanakumar G., et al. “Current status of nanoparticle based imaging agents for early diagnosis of cancer and atherosclerosis”. Journal of Biomedical Nanotechnology 5.1 (2009): 20-35.
- Chen YH., et al. “Methotrexate conjugated to gold nanoparticles inhibits tumor growth in a syngeneic lung tumor model”. Molecular Pharmaceutics 4.5 (2007): 713-722.
- Tseng YC and Huang L. “Self-assembled lipid nanomedicines for siRNA tumor targeting”. Journal of Biomedical Nanotechnology 5.4 (2009): 351-363.
- Andersen AJ., et al. “Complement: Alive and kicking nanomedicines”. Journal of Biomedical Nanotechnology 5.4 (2009): 364-372.
- Asadishad B., et al. “Folate-receptor-targeted delivery of doxorubicin using polyethylene glycol-functionalized gold nanoparticles”. Industrial & Engineering Chemistry Research 49.4 (2010): 1958-1963.
- Erdogan S. “Liposomal nanocarriers for tumor imaging”. Journal of Biomedical Nanotechnology 5.2 (2009): 141-150.
- Hirsch LR., et al. “Nanoshell-mediated near-IR thermal therapy of tumors under magnetic resonance guidance”. Proceedings of the National Academy of Sciences 100.23 (2003): 13549-13554.
- Jordan A., et al.“The effect of thermotherapy using magnetic nanopartcles on rat malignant glioma”. Journal of Neuro-Oncology 78.1 (2006): 7-14.
- Jurgons R., et al. “Drug loaded magnetic nanopartcles for cancer therapy”. Journal of Physics: Condensed Matter 18.38 (2006):2893-2902.
- Zhang L., et al. “Nanoparticles in medicine: Therapeutic applications and developments”. Clinical Pharmacology & Therapeutics 83.5 (2008): 761-769.
- Aravind K., et al. “Rapid green synthesis of silver nanopartcles from root bark extract of Azadirachta indica A. Juss and their application in clinical ultra sound gel”. Annals of drug discovery and biomedical research 1 (2014): 144-154.
- Robert A. Freitas Jr “Nanorobots the size of bacteria might one day roam peoples bodies, rooting out disease organisms and repairing damaged tissue”. Published By The New York Academy Of Sciences. (2000).
- Robert A Freitas Jr “Microbivores: Artificial Mechanical Phagocytes using Digest and Discharge Protocol”. Research Scientist Zyvex Corp March (2001).
- Sondi I and Salopek-Sondi B. “Silver nanoparticles as antimicrobial agent: a case study on E. coli as a model for Gram-negative bacteria”. Journal of Colloid and Interface Science 275.1 (2004): 177-182.
- Gardea-Torresdey JL., et al. “Formation and growth of Au nanoparticles inside live alfalfa plants”. Nano Letters 2.4 (2002): 397-401.
- Gnanadesigan M., et al. “Biosynthesis of silver nanoparticles by using mangrove plant extract and their potential mosquito larvicidal property”. Asian Pacific Journal of Tropical Medicine 4.10 (2011): 799-803.
- Jayaseelan C., et al. “Synthesis of pediculocidal and larvicidal silver nanoparticles by leaf extract from heart leaf moon seed plant Tinospora cordifolia Miers”. Parasitology Research 109.1 (2011): 185-194.
- Jacob SJP., et al. “Synthesis of silver nanoparticles using Piper longum leaf extracts and its cytotoxic activity against Hep-2 cell line”. Colloids and Surfaces A 91 (2012): 212-214.
- Satyavani, K., et al. “Biomedical potential of silver nanoparticles synthesized from calli cells of Citrullus colocynthis (L.) Schrad”. Journal of Nanobiotechnology 9 (2011): 43-51.
- Gurunathan S., et al. “Antiangiogenic properties of silver nanoparticles”. Biomaterials 30.31 (2009): 6341–6350.
- Raghunandan D., et al. “Anti-cancer studies of noble metal nanoparticles synthesized using different plant extracts”. Cancer Nanotechnology 2.1-6 (2011): 57–65.
- Das S., et al. “Biosynthesized silver nanoparticles by ethanolic extracts of Phytolacca decandra, Gelsemium sempervirens, Hydrastis canadensis and Thuja occidentalis induce differential cytotoxicity through G2/M arrest in A375 cells”. Colloids and Surfaces B: Biointerfaces 101(2013): 325–336.
- Alt V., et al. “An in vitro assessment of the antibacterial properties and cytotoxicity of nanoparticle silver bone cement”. Biomaterials 25 (2004): 4383–4391.
- Dipankar C and Murugan S. “The green synthesis, characterization and evaluation of the biological activities of silver nanoparticles synthesized from Iresine herbstii leaf aqueous extracts”. Colloids and Surfaces B: Biointerfaces 98 (2012): 112–119.
- Suman TY., et al. “Biosynthesis, characterization and cytotoxic effect of plant mediated silver nanoparticles using Morinda citrifolia root extract”. Colloids and Surfaces B: Biointerfaces 106 (2013): 74–78.
- Sun RW., et al. “Silver nanoparticles fabricated in Hepes buffer exhibit cytoprotective activities toward HIV-1 infected cells”. Chemical Communications 28 (2005): 5059–5061.
- Daisy P and Saipriya K. “Biochemical analysis of Cassia fistula aqueous extract and phytochemically synthesized gold nanoparticles as hypoglycemic treatment for diabetes mellitus”. International Journal of Nanomedicine 7 (2012): 1189–1202.
- Swarnalatha C., et al. “Evaluation of invitro antidiabetic activity of Sphaeranthus Amaranthoides silver nanoparticles”. Biological and Non-biological Synthesis of Metallic Nanoparticles 2 (2012): 25–29.
- Manikanth SB., et al. “Anti-oxidant effect of gold nanoparticles restrains hyperglycemic conditions in diabetic mice”. Journal of Nanobiotechnology 8 (2010): 77–81.
- Pickup JC., et al. “Nanomedicine and its potential in diabetes research and practice”. Diabetes/Metabolism Research and Reviews 24.8 (2008): 604–610.
- Marambio-Jones C and Hoek EMV. “A review of the antibacterial effects of silver nanomaterials and potential implications for human health and the environment”. Journal of Nanoparticle Research 12.5 (2010): 1531–1551.
- Reichelt KV., et al. “Phytochemical characterization of South African bush tea (Athrixia phylicoides DC)”. South African Journal of Botany 83(2012): 1–8.
- Remédios C., et al. “Environmental nanoparticles interactions with plants: Morphological, physiological, and genotoxic aspects”. Journal of Botany 2012 (2012):1-8.
- Ray PC., et al. “Toxicity and environmental risks of nanomaterials: challenges and future needs”. Journal of environmental science and health. Part C, Environmental carcinogenesis & ecotoxicology reviews 27 (2009): 1-35.
- Li M., et al. “Toxicity of ZnO nanoparticles to Escherichia coli: mechanism and the influence of medium components”. Environmental Science & Technology 45(2011): 1977-1983.
- Sawinska Z., et al. “Wykorzystanie nanocząstek srebra jako fungicydu”. Przemysł Chemiczny 93 (2014): 1472-1474.
- Klaine SJ., et al. “Nanomaterials in the environment: bahavior, fate, bioavailabililty, and effects”. Environmental Toxicology and Chemistry 27.9 (2008): 1825-1851.
- Bhatt I and Tripathi BN. “Interaction of engineered nanoparticles with various components of the environment and possible strategies for their risk assessment”. Chemosphere 82 (2011): 308-317.
- Wallace DR., “Nanotoxicology and metalloestrogens: possible involvement in breast cancer”. Toxics 3.4 (2015): 390-413.
- Seabra AB and Duran N. “Nanotoxicity of metal oxide nanoparticles”. Metals 5.2 (2015): 934-975.
- Reddy PN., et al. “Silver or silver nanoparticles: a hazardous threat to the environment and human health?” Journal of Applied Biomedicine 6 (2008): 117-129.
Citation:
Loutfy H Madkour. “Biogenic– Biosynthesis Metallic Nanoparticles (MNPs) for Pharmacological, Biomedical and Environmental
Nanobiotechnological Applications”. Chronicles of Pharmaceutical Science 2.1 (2018): 384-444.
Copyright: © 2018 Loutfy H Madkour. This is an open-access article distributed under the terms of the Creative Commons Attribution License, which permits unrestricted use, distribution, and reproduction in any medium, provided the original author and source are credited.