Editorial
Volume 2 Issue 1 - 2018
Novel Anti-Cancer Drugs Approved in 2017
Drug Safety Assessment, Novel Drug Discovery and Development, Lupin Limited (Research Park), 46A/47A, Nande Village, Taluka-Mulshi, Pune - 412115, India
*Corresponding Author: Varun Ahuja, Drug Safety Assessment, Novel Drug Discovery and Development, Lupin Limited (Research Park), 46A/47A, Nande Village, Taluka-Mulshi, Pune - 412115, India.
Received: February 08, 2018; Published: February 16, 2018
Keywords: Anti-cancer; Drug; FDA; Mechanism of action
In 2017, CDER (Food and Drug Administration, USA) approved 46 new drugs, out of which 12 (26%) were anti-cancer drugs [1]. Here, we review the mechanism of action of various approved anti-cancer drugs (Table 1).
S. No. | Drug (Brand Name) | Approved use/Indication | Class |
1 | Acalabrutinib (Calquence) | Treatment of adult patients with mantle cell lymphoma (MCL) who have received at least one prior therapy. | Kinase inhibitor |
2 | Abemaciclib (Verzenio) | Treatment of adult patients with HR (hormone receptor) positive, HER2 (human epidermal growth factor receptor) ‐negative advanced or metastatic breast cancer with disease progression following endocrine therapy and prior chemotherapy in the metastatic setting. | Kinase inhibitor |
3 | Copanlisib dihydrochloride (Aliqopa) |
Treatment of patients with relapsed follicular lymphoma who have received at least two prior therapies. | Kinase inhibitor |
4 | Inotuzumab ozogamicin (Besponsa)a |
Relapsed or refractory B-cell precursor acute lymphoblastic leukemia(ALL) | Antibody-Drug Conjugate |
5 | Enasidenib mesylate (Idhifa) | Treatment of patients with relapsed or refractory acute myeloid leukemia with an IDH2 (isocitrate dehydrogenase-2) mutation | Isocitrate dehydrogenase-2 inhibitor |
6 | Neratinib maleate (Nerlynx) | Treatment of adult patients with early stage ERBB2-positive breast cancer who have received prior adjuvant trastuzumab-based therapy. | Kinase inhibitor |
7 | Durvalumab (Imfinzil) a | Advanced or metastatic urothelial carcinoma | Programmed death-ligand 1 (PD-L1) blocking antibody |
8 | Midostaurin (Rydapt) | Advanced systemic mastocytosis and FLT3 mutation positive acute myeloid leukemia | Kinase inhibitor |
9 | Brigatinib (Alunbrig) | Treatment of patients with anaplastic lymphoma kinase (ALK)-positive metastatic non-small cell lung cancer (NSCLC) who have progressed on or are intolerant to crizotinib. | Kinase inhibitor |
10 | Niraparib (Zejula) | Maintenance treatment of adult patients with platinum‐sensitive recurrent epithelial ovarian, fallopian tube, or primary peritoneal cancer who are in response to platinum‐based chemotherapy. | Poly(ADP‐ribose) polymerase (PARP) inhibitor |
11 | Avelumab (Bavencio)a | Metastatic Merkel cell carcinoma (MCC) | Programmed death-ligand 1 (PD-L1) blocking antibody |
12 | Ribociclib (Kisqali) | Indicated in combination with letrozole for the treatment of postmenopausal women with hormone receptor (HR)-positive, human epidermal growth factor receptor 2 (HER 2)-negative advanced or metastatic breast cancer as initial endocrine-based therapy. | Kinase inhibitor |
a: Biologic therapy
Table 1: New approved anti-cancer drugs in 2017.
Table 1: New approved anti-cancer drugs in 2017.
Kinase Inhibitors: The various kinase inhibitors approved included: acalabrutinib, abemaciclib, copanlisib dihydrochloride, neratinib, brigatinib and ribociclib.
Acalabrutinib is an inhibitor of Bruton tyrosine kinase (BTK) [Figure 1]. BTK, also known as agammaglobulinemia tyrosine kinase (ATK) or B-cell progenitor kinase (BPK), is a non-receptor tyrosine kinase that was initially identified as the defective protein in human X-linked agammaglobulinemia (XLA) [2]. BTK is a signaling molecule of the B-cell antigen receptor (BCR) and cytokine receptor pathways that is expressed in B-cells, myeloid cells, mast cells, and platelets. BTK’s role in signaling through the B-cell surface receptors results in activation of pathways necessary for B-cell trafficking, chemotaxis, and adhesion. Chronic activation of the BCR pathway is involved in the proliferation and cell survival of various B-cell malignancies. In steady state conditions, BTK is predominantly cytosolic, unphosphorylated and catalytically inactive. BTK activation is a complex process and a critical step in this process requires translocation of BTK to the plasma membrane [3].
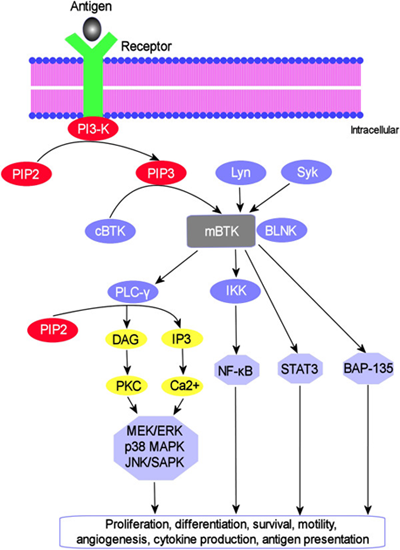
Figure 1: BTK signaling pathway. BTK translocates to the plasma membrane by interacting with PIP3 to become membrane-bound where it undergoes sequential activation through trans-phosphorylation by Lyn and Syk kinases, followed by autophosphorylation. The downstream substrates of activated BTK and their associated signaling cascades are indicated. [Reprinted from: Akinleye., et al. Journal of Hematology and Oncology 6.59 (2013): 1-9]
Abemaciclib and Ribociclib have activity against cyclin‐dependent kinase 4 (CDK4) and CDK6 [Figure 2]. The mammalian cell cycle involves sequential activation of related heterodimeric protein kinases, including a catalytic subunit, the cyclin-dependent kinase (CDK), and a regulatory subunit, the cyclin. CDK4 and CDK6 are activated by the D-type cyclins and implicated in regulating the early phases of the cell cycle, especially during exit from quiescence. CDK4/6-cyclin D heterodimeric kinases promote re-entry into the cycle by initiating phosphorylation of the retinoblastoma protein family, Rb, p107, and p130. Rb phosphorylation results in the liberation of transcription factors, e.g., members of the E2F family, which are bound to the hypophosphorylated Rb proteins in non-proliferation cells. These transcription factors are responsible for directing the expression of genes essential for advancing cells through the S phase of the cell cycle. The expression of cyclins, such as Cyclin-D1, determines, in part, the extent to which their CDK counterparts are activated. Cyclin expression is regulated by gene transcription downstream of cellular signaling pathways, such as those activated by mitogenic stimuli. In addition, regulation of the cell cycles includes cellular inhibitors of CDKs and cyclin-CDK complexes, such as p27/Kip1 or p16/INK4a, and other signaling molecules. There is some redundancy of the activities among the different types and pairings of cyclin-CDKs, such that loss or inhibition of one or more of these complexes in a certain cell type may be compensated for by another cyclin-CDK or through some other compensatory mechanism and cell division may not be completely blocked. The expression pattern of cyclins, CDKs and cyclin-CDK inhibitors in various cell types may determine whether a CDK has a redundant or non-redundant role in a specific cell type [4]. In estrogen receptor‐positive (ER+) breast cancer, ER activation promotes cyclin D1 transcription and activation of CDK4/6‐Rb pathway, leading to uncontrolled cell proliferation.
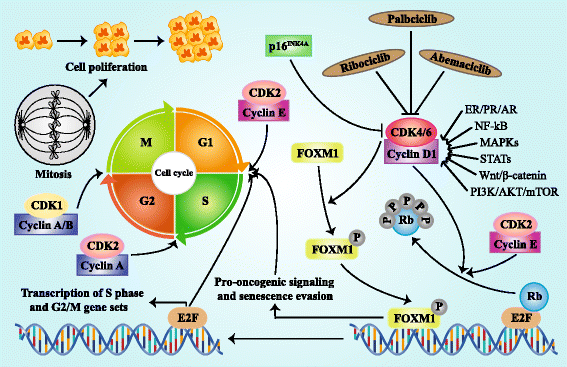
Figure 2: Regulation and function of CDK4/6 in cell cycle progression. Active complex of CDK4/6 and cyclin D phosphorylates and inactivates RB protein and then releases transcription factor E2F, triggering the up-regulation of E2F-responsive gene which promotes cell proliferation with cell cycle G1/S transition. The combination of CDK4/6 and cyclin D can also phosphorylates transcription factor FOXM1, resulting in the FOXM1-dependent expression of gene which protects cancer cells from cell cycle block. The kinase activity of CDK4/6 is suppressed by p16INK4A and pharmacologic CDK4/6 inhibitors. Cyclin D is regulated by multiple pathways such as ER/PR/AR, NF-kB, MAPKs, STATs, Wnt/β-catenin, and PI3K/AKT/mTOR. Besides, CDK2/cyclin E also participates in the RB phosphorylation. CDK2/cyclin A complex increases in stages S, G2, and M, while CDK1/Cyclin A/B complex mediates the transition from G2 to M stage [Reprinted from: Xu., et al. Journal of Hematology and Oncology 10.97 (2017): 1-12]
Copanlisib is an inhibitor of phosphatidylinositol-3-kinase (PI3K) [Figure 3]. PI3K is a lipid kinase involved in activation of the PI3K/3-phosphoinositide-dependent protein kinase-1 (PDK1) and AKT pathways. PI3K also modulates guanosine triphosphate exchange factor (GEPFs) activity through generation of phosphoinositol-3-phosphate (PIP3). The PI3K-α and PI3K-δ isoforms are expressed in malignant B cells [5].
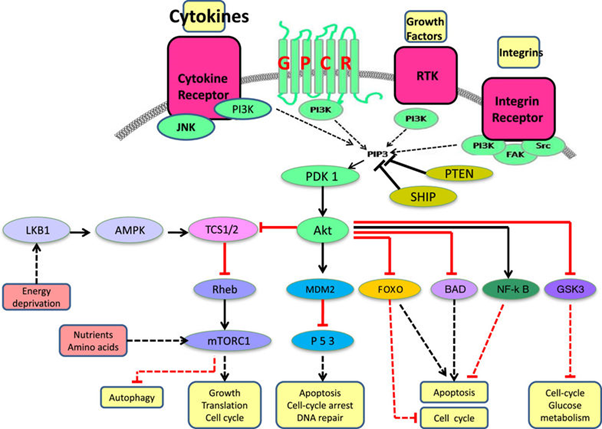
Figure 3: Schematic representation of the PI3K signaling pathway. Four major extracellular signals, growth factors, cytokines, hormones/chemokines, and integrins, activate PI3K, which transmit the signals through appropriate pathways to control diverse cellular processes, including cell cycle, apoptosis, DNA repair, senescence, angiogenesis, cellular metabolism, autophagy, and motility. The multiple effector kinase pathways activated by PI3K are highlighted in the figure. [Reprinted from: Akinleye., et al. Journal of Hematology and Oncology 6.88 (2013): 1-17]
Neratinib is a new molecular entity and an orally available small molecule kinase inhibitor [Figure 4]. It irreversibly binds to Epidermal Growth Factor Receptor (EGFR), Human Epidermal Growth Factor Receptor 2 (HER2), and HER4. The HER signaling pathways are normally involved in regulation of cell growth and survival as well as adhesion, migration, differentiation, and other cellular responses. There are four members of the family, including EGFR/HER1, HER2, HER3, and HER4 (also called erbB-1, erbB-2, erbB-3, and erbB-4, respectively) [6].
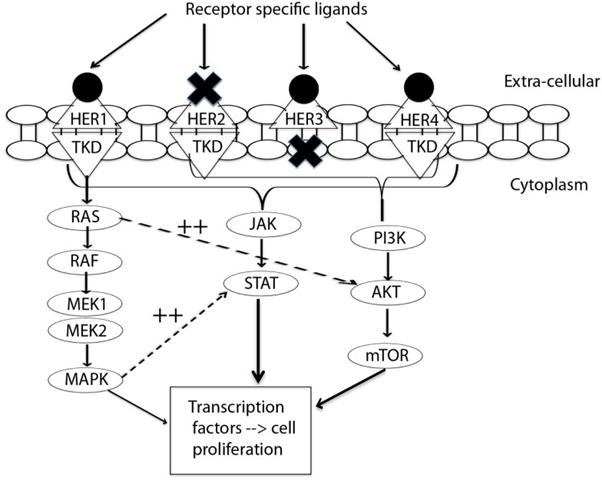
Figure 4: Signal transduction by HER-family. This figure summarizes the interplay between three pathways: MAPK, P13K/AKT, and JAK/STAT. MAPK dramatically enhances transcriptional activation by STAT (11). EGFR/HER1 cannot directly activate the P13K/AKT pathway, but it couples to the ras/MAPK pathway as well as to the ras/PI3K/AKT pathway. This interplay of pathways forms the source of by-pass resistance to EGFR TKIs. TKD, tyrosine kinase domain; MEK, mitogen activated protein kinase kinase; MAPK, mitogen activated protein kinase; PI3K, phosphoinositide 3-kinase; mTOR, mammalian target of rapamycin; JAK, janus kinase; STAT, signal transducer and activator of transcription. [Reprinted from: Wang., et al. Frontiers in Oncology 5.2 (2015): 1-12]
Brigatinib is a tyrosine kinase inhibitor that has activity against multiple kinases including anaplastic lymphoma kinase (ALK), ROS1, insulin-like growth factor receptor-1 (IGF-1R), and FLT-3 as well as EGFR.
Midostaurin is a small molecule inhibitor of fms-like tyrosine kinase-3 (FLT3) and tyrosine kinase KIT (KIT) [Figure 5]. FLT3 (FMS-like tyrosine kinase 3) belongs to a type III receptor tyrosine kinase together with KIT, FMS and PDGF-receptor. FLT3 expresses on the surface of normal hematopoietic stem/progenitor cells. FLT3 ligand (FL) is expressed by bone marrow stroma cells, and FL-FLT3 interaction plays an important role in the survival, proliferation and differentiation of normal hematopoietic stem/progenitor cells. In addition, FLT3 expresses in most AML (acute myeloid leukemia) and ALL (acute lymphocytic leukemia) cells, and FL-stimulation enhances proliferation and reduces apoptosis of leukemia cells [7].
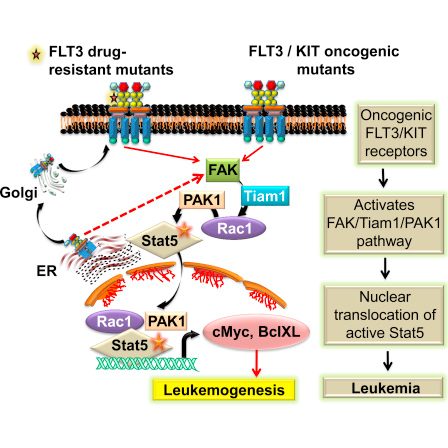
Figure 5: FLT3/KIT pathway. [Reprinted from: Chatterjee., et al. Cell Reports 9.4 (2014): 1333-1348]
Isocitrate dehydrogenase 2 (IDH2) inhibitor: Enasidenib is an isocitrate dehydrogenase 2 (IDH2) inhibitor. The IDH enzymes catalyze the oxidative decarboxylation of isocitrate to alpha-ketoglutarate (α-KG), producing nicotinamide adenine dinucleotide phosphate (NADPH) in the process via the citric acid cycle. Some mutant forms of IDH enzymes catalyze the reduction of α-KG to an oncometabolite known as 2-hydroxyglutarate (2-HG), while consuming NADPH [8].
Poly (ADPribose) polymerase (PARP) inhibitor: Niraparib is a new molecular entity and inhibitor of poly(ADP-ribose) polymerase (PARP) 1 and 2 [Figure 6]. PARP is a family of proteins involved in DNA repair. Inhibition of PARP enzymatic activity can result in DNA damage, apoptosis and cell death. There about 17 proteins in this family out of which the primary nuclear PARPs are PARP-1, PARP-2, PARP-3, and tankyrases 1 and 2 (PARP-5a and -5b). The PARP family members are known to engage in a wide range of cellular activities, for example, DNA repair, transcription, cellular signaling, cell cycle regulation and mitosis amongst others [9].
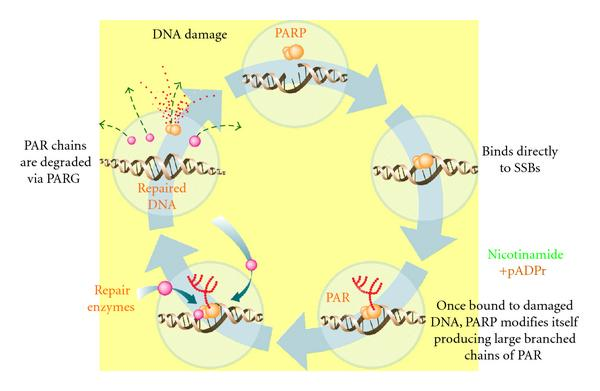
Figure 6: PARP-1 mediates the repair of SSBs via the activation and recruitment of repair enzymes. Clockwise: PARP-1 binds to the DNA adjacent to the damage detecting and signaling the presence of an SSB. Once bound, PARP-1 catalyzes the cleavage of the coenzyme nicotinamide adenine dinucleotide (NAD+) into nicotinamide and ADP-ribose to produce highly charged branched chains of poly(ADP-ribose) (PAR). Then DNA ligase III (LigIII), DNA polymerase beta (polβ), and scaffolding proteins such as x-ray repair complementing gene 1 (XRCC1) are recruited to the site of damage, to repairing the damaged DNA. After repair, the PAR chains are degraded via PAR glycohydrolase (PARG). [Reprinted from: Gien and Mackay. Journal of Oncology. (2010). Article ID 151750]
Antibody-Drug Conjugate: Inotuzumab ozogamicin is an antibody-drug conjugate (ADC) consisting of a humanized CD22-directed IgG4 monoclonal antibody (inotuzumab, G544) and a semi-synthetic derivative of the cytotoxic natural product γ-calicheamicin (N-acetyl-γ-calicheamicin dimethylhydrazide or N-Ac-γ-calicheamicin DMH), with a small molecule-to-antibody ratio of approximately 6:1 [Figure 7]. Antibody-drug conjugates (ADCs) consist of cytotoxic drugs covalently linked to monoclonal antibodies directed to antigens differentially overexpressed in tumor cells. These loaded antibodies are expected to selectively deliver lethal cargoes to tumor cells and provide sustained clinical benefit to pre-selected cancer patients while, at the same time, minimizing systemic toxicity. The combination of MAb and cytotoxin lends itself to synergism. MAbs may act as target-selective anti-cancer agents by number of different mechanisms: complement-dependent cytolysis (CDC), antibody-dependent cellular cytotoxicity (ADCC), antibody dependent phagocytosis, induction of apoptosis, depletion of circulating tumor cells, and interference with cellular signaling associated with proliferative activity [10].
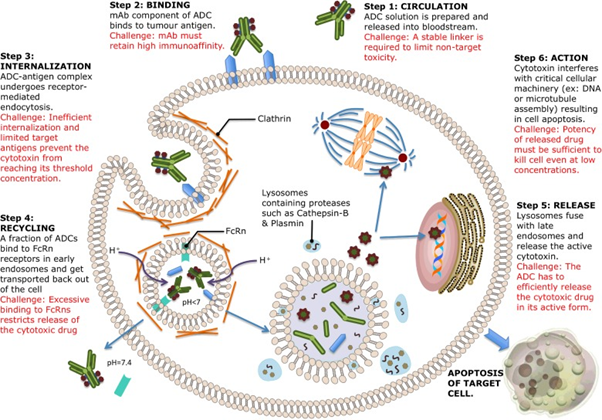
Figure 7: Mechanism of action of ADCs [Reprinted from: Peters and Brown. Bioscience Reports 35.4 (2015): 1-20]
Programmed death-ligand 1 (PD-L1) blocking antibody: Approved PD-L1 blocking antibodies included durvalumab and avelumab.
Durvalumab and Avelumab are human IgG1 monoclonal antibodies that bind to human programmed death ligand 1 (PD-L1), thereby inhibiting the interaction of PD-L1 with PD-1 and B7-1 receptors [Figure 8]. PD-L1 is a member of the B7 family of ligands that regulate the activation of T cells through binding to PD1 and CD80 receptors. PD-L1 is expressed on cells of the immune system and on many human tumors at high frequency. In the tumor microenvironment, PD-L1 expressed on tumor cells binds to PD-1 on activated T cells and delivers inhibitory signal resulting in inhibition of T-cell activation; thereby preventing killing of target tumor cells and immune elimination of tumors. The expression of PD-L1 is associated with poor prognosis for patient survival. In nonclinical studies, it was reported that blocking PD-L1 interaction with its receptors releases inhibition of the immune response, resulting in enhanced immune surveillance and response, including immune-mediated anti-tumor activity [11]. An additional mechanism of action proposed for avelumab is antibody dependent cell mediated cytotoxicity (ADCC) which is thought to occur as a result of the engagement of fragment crystalline receptors on cytotoxic natural killer cells and macrophages.
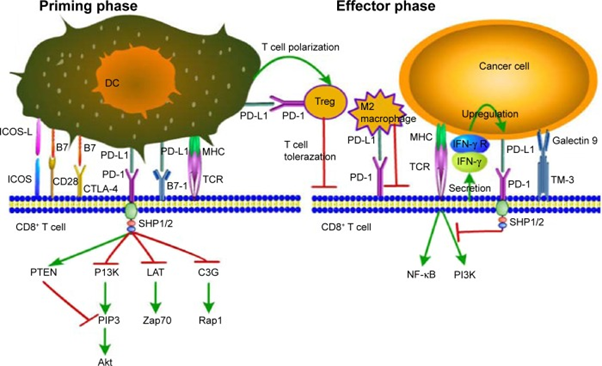
Figure 8: PD-1/PD-L1 signaling: decreased CD8+ T cell proliferation, survival, and cytokine production. (DC, dendritic cell; Treg, regulatory T cell; ICOS, inducible costimulator; ICOS-L, inducible costimulator-ligand; CD28, cluster of differentiation 28; CTLA-4, cytotoxic T lymphocyte-associated antigen-4; PD-L1, programmed death-ligand 1; PD-1, programmed death-1; MHC, major histocompatibility complex; TCR, T cell receptor; IFN-γ, interferon-γ; IFN-γR, interferon-γ Receptor) [Reprinted from: Wang., et al. Onco Targets and Therapy, 9 (2016): 5023-5039]
References
- Mullard A. “2017 FDA drug approvals”. Nature Reviews Drug Discovery 17 (2018): 81-85.
- Tsukada S., et al. “Deficient expression of a B cell cytoplasmic tyrosine kinase in human X-linked agammaglobulinemia”. Cell 72.2 (1993): 279-290.
- Varnai P., et al. “Phosphatidylinositol 3-kinase-dependent membrane association of the Bruton’s tyrosine kinase pleckstrin homology domain visualized in single living cells”. The Journal of Biological Chemistry 274.16 (1999): 10983-10989.
- Xu H., et al. “Recent advances of highly selective CDK4/6 inhibitors in breast cancer”. Journal of Hematology and Oncology 10.97 (2017): 1-12.
- Akinleye A., et al. “Phosphatidylinositol 3-kinase (PI3K) inhibitors as cancer therapeutics”. Journal of Hematology and Oncology 6.88 (2013): 1-17.
- Wang X., et al. “The potential of panHER inhibition in cancer”. Frontiers in Oncology 5.2 (2015): 1-12.
- Kiyoi H. “FLT3 inhibitors: recent advances and problems for clinical application.” Nagoya Journal of Medical Science 77(1-2) (2015): 7-17.
- Stein M. “IDH2 inhibition in AML: Finally progress?” Best Practice and Research Clinical Hematology 28 (2-3) (2015): 112-115.
- Anwar M., et al. “PARP inhibitors”. Hereditary Cancer in Clinical Practice 13.4 (2015): doi: 10.1186/s13053-014-0024-8.
- Hamilton GS. “Antibody-drug conjugates for cancer therapy: The technological and regulatory challenges of developing drug-biology hybrids”. Biologicals 43.5 (2015): 318-332.
- Wang X., et al. “PD-L1 expression in human cancers and its association with clinical outcomes”. Onco Targets and Therapy 12.9 (2016): 5023-5039.
- Akinleye A., et al. “Ibrutinib and novel BTK inhibitors in clinical development”. Journal of Hematology and Oncology 6.59 (2013): 1-9.
- Chatterjee A., et al. “Regulation of Stat5 by FAK and PAK1 in oncogenic FLT3- and KIT-driven leukemogenesis”. Cell Reports 9.4 (2014): 1333-1348.
- Gien LT and Mackay HJ. “The emerging role of PARP inhibitors in the treatment of epithelial ovarian cancer”. Journal of Oncology2010 (2010): 6.
- Peters C and Brown S. “Antibody-drug conjugates as novel anti-cancer therapeutics”. Bioscience Reports 35.4 (2015): 1-20.
Citation:
Varun Ahuja. “Novel Anti-Cancer Drugs Approved in 2017”. Chronicles of Pharmaceutical Science 2.1 (2018): 445-452.
Copyright: © 2018 Varun Ahuja. This is an open-access article distributed under the terms of the Creative Commons Attribution License, which permits unrestricted use, distribution, and reproduction in any medium, provided the original author and source are credited.