Research Article
Volume 2 Issue 4 - 2018
Changes in Resting Metabolism, Body Fat, and Fat-Free Mass among College Students in Response to Novel High-Intensity Weight-Supported Exercise
1Department of Natural Sciences, Tiffin University, USA
2Department of Human Services, University of Cincinnati, USA
3Department of Rehabilitation Sciences, University of Cincinnati, USA
2Department of Human Services, University of Cincinnati, USA
3Department of Rehabilitation Sciences, University of Cincinnati, USA
*Corresponding Author: Matthew D McCabe, Department of Natural Sciences, Tiffin University, USA.
Received: July 26, 2018; Published: August 08, 2018
Abstract
Purpose: The aim of this pilot study was to examine the impact of high-intensity low-volume exercise training (HILVET) utilizing a novel weight-supported kinetic chain resistance training (WSKCRT) modality on factors related to long-term weight management through measurements of resting metabolic rate (RMR), fat-free mass, fat mass, and body fat percentage (BF%).
Methods: Six recreationally active undergraduate college students completed high-intensity low-volume WSKCRT on the FinisherTM, a novel WSKCRT modality, three days per week for six weeks. Training consisted of performing 4-9 intervals of upper body exercise, on the FinisherTM, for 30 seconds at 90% of heart rate reserve (HRR) followed by 60 seconds of treadmill exercise at 40% of HRR.
Results: Significant reductions in fat mass (19.2 ± 9.7 to 16.6 ± 8.3 kg; p = 0.010; d = 0.288) and BF% (23.1 ± 8.7 to 20.3 ± 7.6 %; p = 0.004; d = 0.343) were observed. Changes in RMR (1,859.6 ± 620.6 to 2,271.6 ± 761.6 kcal/day; p = 0.336) and fat-free mass (60.03 ± 13.6 to 62.7 ± 13.7 kg; p = 0.028) failed to reach statistical significance.
Conclusions: These results indicate the potential efficacy of HILVET, using the FinisherTM, as a time-efficient tool for managing body weight in a recreationally active college population. Further testing of this intervention is required.
Keywords: High-intensity low-volume exercise training; Body fat percentage; Resting metabolic rate
Introduction
According to body mass index (BMI) classifications, national data suggest that approximately 69% of adults in the United States are classified as being overweight or obese [1]. Even more compelling, the National College Health Assessment II [2], conducted by the American College Health Association reported that 35% of all college students are classified as overweight or obese. This poses a long-term health concern given the known contribution of poor body composition to a plethora of chronic diseases [1, 3-5]. Exercise is a commonly recommended treatment strategy for managing and preventing obesity [6].
However, the majority of adults and approximately half of college-aged adults do not adhere to the minimum recommended exercise dose for improving health and preventing weight gain, citing a lack of time as a common barrier [7]. Therefore, much attention has been given to high-intensity low-volume exercise training (HILVET) as a means of improving body composition [8,9]. HILVET can be defined as exercise that involves repeated bouts of intense exercise separated by periods of active or passive rest with exercise sessions lasting 10-30 minutes [10].
HILVET has been shown to elicit improvements of key contributing factors in the development of poor body composition including fat mass, fat-free mass, and body fat percentage (BF%) [9,11]. Trapp., et al. [11] had participants perform 8 seconds of high-intensity cycling followed by 12 seconds of low-intensity cycling repeatedly for 20 minutes. After 45 exercise sessions, these subjects had significantly improved fat mass and fat-free mass in comparison to subjects who completed steady state exercise training.
Heydari., et al. [9] conducted a similar study in which participants completed repeated bouts of 8 seconds of high-intensity cycling performed at 80-90% of peak heart rate followed by 12 seconds of low-intensity cycling for 20 minutes. This intervention was shorter than Trapp., et al. [11] lasting 12 weeks in comparison to 15 weeks. The subjects in Heydari., et al. [9] saw an average decrease in fat mass of 2 kg and an average increase in fat-free mass 1.2 kg, both of which were significantly different than a non-exercise control group.
Repeated, transient, elevations in resting metabolic rate (RMR) is a proposed mechanism for inducing positive changes in fat mass and BF% in response to exercise [12]. A notable determinant of RMR is the phenomena of excess post-exercise oxygen consumption, which represents the elevation in oxygen consumption up to 48 hours after a bout of exercise [13]. This phenomenon occurs due to a myriad of factors including elevated body temperature, increased circulation of catecholamines, increased presence of thyroid hormones, the replenishment of phosphocreatine stores, and the removal of blood lactate, all of which results in, and are reflective of, an elevation in RMR [14-16]. Additionally, there is a direct relationship between fat-free mass and RMR [17,18]. With this knowledge, it is important for exercise protocols to induce positive changes in fat-free mass when attempting to favorably influence body composition. However, studies examining changes in RMR in response to HILVET are sparse and those that have examined RMR have revealed non-significant changes [9].
A specific challenge of designing HILVET programs is prescribing protocols that can be modeled by participants in non-laboratory settings. Typically, HILVET protocols require participants to perform maximal or near-maximal bouts of exercise, often involving load-bearing movements [19-22]. These high-intensity bursts of exercise may not be transferrable to practical settings. Therefore, it is important for future researchers to focus on the evaluation of novel and practical HILVET protocols that are translatable to professional application. Standing weight-supported kinetic chain resistance training (WSKCRT) is a method of training shown to improve power in the muscles of the pelvis, spine and trunk resulting in enhanced sport-specific performance [23].
However, incorporating WSKCRT into a HILVET protocol has only been evaluated using weight-supported treadmills, which do not incorporate the limbs of the upper body [24]. Moreover, its effects on factors contributing to the development of poor body composition are unknown. The FinisherTM (Finishing Fitness, West Harrison, IN, USA), is a novel WSKCRT modality, that requires its users to repetitively push and pull hand weights across a horizontal surface (Figure 1). The nature of this movement invokes both aerobic and resistance stimuli. Having participants perform short high-intensity intervals of upper body movements on the FinisherTM while isometrically contracting the muscles of the lower body and core may be an optimal and practical stimulus to positively influence RMR, fat-free mass, fat mass, and BF%.
Taking into consideration a common barrier to exercise participation in college students, the efficacy of HILVET to evoke favorable changes in body composition in less time than traditional steady state protocols, and the need to evaluate novel HILVET protocols that are more translatable to practical application, this pilot study sought to evaluate the effectiveness of a novel high-intensity WSKCRT program among recreationally active college students. With the role that RMR plays in eliciting improvements in fat mass and BF%, coupled with the direct relationship between fat-free mass and RMR, the outcomes examined in this study included RMR, fat-free mass, fat mass, and BF%. We hypothesized that six weeks of high-intensity WSKCRT would significantly increase RMR and fat-free mass in addition to significantly reducing fat mass and BF%.
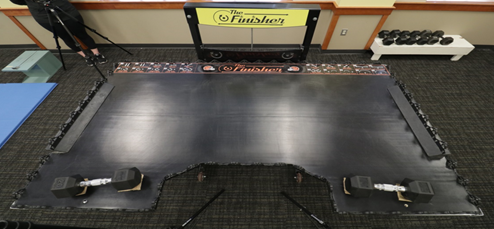
Figure 1: Overhead view of the surface of the FinisherTM. Participants vigorously
pushed and pulled dumbbells across the horizontal surface to maintain 90% HRR.
Materials and Methods
Participants
Six undergraduate college students, three males and three females, participated in the study. Table 1 shows the participants’ baseline characteristics for age, body mass, and height. All participants self-reported exercising at a moderate intensity, three days per week, over the previous three months. Inclusion criteria was based on the following: 1) self-reported exercising at a moderate intensity, three days per week, over the previous three months; 2) current undergraduate college student; 3) between the ages of 18 and 25 years old. All volunteers were screened using the Physical Activity Readiness Questionnaire for everyone [25] and were excluded from the study if they reported having a medical condition that would require medical clearance prior to starting an exercise program.
Six undergraduate college students, three males and three females, participated in the study. Table 1 shows the participants’ baseline characteristics for age, body mass, and height. All participants self-reported exercising at a moderate intensity, three days per week, over the previous three months. Inclusion criteria was based on the following: 1) self-reported exercising at a moderate intensity, three days per week, over the previous three months; 2) current undergraduate college student; 3) between the ages of 18 and 25 years old. All volunteers were screened using the Physical Activity Readiness Questionnaire for everyone [25] and were excluded from the study if they reported having a medical condition that would require medical clearance prior to starting an exercise program.
The participants were instructed to refrain from additional exercise beyond the intervention and maintain their current diets; however records of the participants’ exercise beyond the intervention and diets were not taken. All participants were informed of all testing and intervention procedures along with the potential risks associated with participation. Informed consent was obtained from all participants prior to pre-intervention testing. The present study was approved by the University’s Institutional Review Board for studies involving human subjects.
Procedures
Seven to ten days prior to pre-intervention testing, participants completed a familiarization session. The familiarization session consisted of participants completing the pre- and post-intervention RMR test and cardiorespiratory exercise testing procedure in order to limit learning effects. Additionally, during the familiarization session, participants completed one to two 30 second intervals of HILVET, at an estimated intensity of 90% of heart rate reserve (HRR), performed on the FinisherTM. The cardiorespiratory test completed was a Bruce Treadmill Protocol. The pre- and post-intervention assessment for fat mass, fat-free mass, and BF% was a seven-site skinfold assessment and this was not conducted during the familiarization session.
Seven to ten days prior to pre-intervention testing, participants completed a familiarization session. The familiarization session consisted of participants completing the pre- and post-intervention RMR test and cardiorespiratory exercise testing procedure in order to limit learning effects. Additionally, during the familiarization session, participants completed one to two 30 second intervals of HILVET, at an estimated intensity of 90% of heart rate reserve (HRR), performed on the FinisherTM. The cardiorespiratory test completed was a Bruce Treadmill Protocol. The pre- and post-intervention assessment for fat mass, fat-free mass, and BF% was a seven-site skinfold assessment and this was not conducted during the familiarization session.
Seven days after the familiarization and 72 prior to the first exercise training session, pre-intervention testing was completed. This testing session consisted of subjects completing the RMR test, the Bruce Treadmill Protocol, and anthropomorphic measures. These same testing procedures were completed, in the same order, post-intervention approximately 72 hours after the final exercise training session.
Resting Metabolic Rate Testing
Participants were asked to refrain from consuming foods and beverages, except for water, during the 12 hours prior to RMR testing. Additionally, all participants were asked to abstain from alcohol and caffeine consumption during the 24 hours prior to testing. Also, all participants were asked to refrain from exercise or recreational activity during the 72 hours prior to RMR testing. RMR testing took place 72 hours pre-intervention and 72 hours after the final exercise session. On the day of RMR testing, participants were instructed to only walk at light paces, avoid hills and stairs, and not carry any excess weight in the form of back packs or hand bags.
Participants were asked to refrain from consuming foods and beverages, except for water, during the 12 hours prior to RMR testing. Additionally, all participants were asked to abstain from alcohol and caffeine consumption during the 24 hours prior to testing. Also, all participants were asked to refrain from exercise or recreational activity during the 72 hours prior to RMR testing. RMR testing took place 72 hours pre-intervention and 72 hours after the final exercise session. On the day of RMR testing, participants were instructed to only walk at light paces, avoid hills and stairs, and not carry any excess weight in the form of back packs or hand bags.
Upon arrival to the laboratory, participants were asked to lie in the supine position, in a dark quiet room, for 30 minutes. The participants were instructed to remain conscious and avoid fidgeting and talking. Next, continuous measures of carbon dioxide and oxygen gasses were averaged and recorded every 20 seconds for 15 minutes using open-circuit spirometry (True One 2400, Parvo Medics Inc., and Sandy, UT, USA). Averages of the collected carbon dioxide and oxygen values were used to calculate 24-hour RMR using the modified Weir equation [26]. The modified Weir equation is as follows: RMR = [3.94 (volume of oxygen consumption in milliliters/minute) + 1.1 (volume of carbon dioxide elimination in milliliters/minute)] x 1.44.
Anthropomorphic Assessment
Fat-free mass, fat mass, and body fat percentage were determined using the 7-site skinfold method. The same investigator performed all skinfold assessments, using a Lange Skinfold Caliper (Beta Technology, Santa Cruz, CA, USA). Skinfold sites included the mid-triceps, mid-pectoral, subscapula, mid-axilla, abdomen, suprailliac crest, and the mid-thigh. All skinfolds were collected from the right side of the body. A total of three skinfolds were taken and averaged for each site. The skinfold averages were summed and inserted into sex-specific body density equations [27]. Body density was then converted to an estimate of BF% using the Siri equation [28]. From BF% estimates, fat-free mass and fat mass were calculated based on body weight.
Fat-free mass, fat mass, and body fat percentage were determined using the 7-site skinfold method. The same investigator performed all skinfold assessments, using a Lange Skinfold Caliper (Beta Technology, Santa Cruz, CA, USA). Skinfold sites included the mid-triceps, mid-pectoral, subscapula, mid-axilla, abdomen, suprailliac crest, and the mid-thigh. All skinfolds were collected from the right side of the body. A total of three skinfolds were taken and averaged for each site. The skinfold averages were summed and inserted into sex-specific body density equations [27]. Body density was then converted to an estimate of BF% using the Siri equation [28]. From BF% estimates, fat-free mass and fat mass were calculated based on body weight.
Cardiorespiratory Fitness Testing
Cardiorespiratory fitness was assessed via a maximal Bruce Treadmill Protocol with continuous heart rate monitoring. The Bruce Treadmill Protocol was terminated and the participant was excluded from the study if any major musculoskeletal, pulmonary, or cardiovascular symptoms occurred. Upon volitional fatigue, participants’ maximal heart rates were recorded and used to determine appropriate exercise intensities during the intervention.
Cardiorespiratory fitness was assessed via a maximal Bruce Treadmill Protocol with continuous heart rate monitoring. The Bruce Treadmill Protocol was terminated and the participant was excluded from the study if any major musculoskeletal, pulmonary, or cardiovascular symptoms occurred. Upon volitional fatigue, participants’ maximal heart rates were recorded and used to determine appropriate exercise intensities during the intervention.
Training
The training intervention was carried out over six weeks. Participants completed high-intensity WSKCRT three days per week and accumulated a total of 18 exercise sessions. Exercise sessions consisted of 30 second bouts of exercise on the FinisherTM at 90% of heart rate reserve (HRR). HRR was calculated as follows: [(maximal heart rate achieved during the Bruce Treadmill Protocol - resting heart rate) x (0.90)] + resting heart rate. To perform high-intensity WSKCRT on the FinisherTM, participants vigorously pushed and pulled dumbbells across the horizontal platform of the Finisher (Figure 2). Participants self-selected the weight of the dumbbells based on their ability to maintain 90% HRR. The dumbbells ranged from 9.1-15.9 kg. Participants were instructed to maintain a 15 degree knee bend with an upright torso (Figure 2). After a bout of high-intensity WSKCRT exercise on the FinisherTM, participants then walked/jogged on a treadmill for 60 seconds at 40% HRR. This process was completed in intervals starting with four and progressing to nine intervals per session. All heart rates were monitored using a Polar T31 Non-coded Chest Transmitter (Polar Electro, Kempele, Finland).
The training intervention was carried out over six weeks. Participants completed high-intensity WSKCRT three days per week and accumulated a total of 18 exercise sessions. Exercise sessions consisted of 30 second bouts of exercise on the FinisherTM at 90% of heart rate reserve (HRR). HRR was calculated as follows: [(maximal heart rate achieved during the Bruce Treadmill Protocol - resting heart rate) x (0.90)] + resting heart rate. To perform high-intensity WSKCRT on the FinisherTM, participants vigorously pushed and pulled dumbbells across the horizontal platform of the Finisher (Figure 2). Participants self-selected the weight of the dumbbells based on their ability to maintain 90% HRR. The dumbbells ranged from 9.1-15.9 kg. Participants were instructed to maintain a 15 degree knee bend with an upright torso (Figure 2). After a bout of high-intensity WSKCRT exercise on the FinisherTM, participants then walked/jogged on a treadmill for 60 seconds at 40% HRR. This process was completed in intervals starting with four and progressing to nine intervals per session. All heart rates were monitored using a Polar T31 Non-coded Chest Transmitter (Polar Electro, Kempele, Finland).
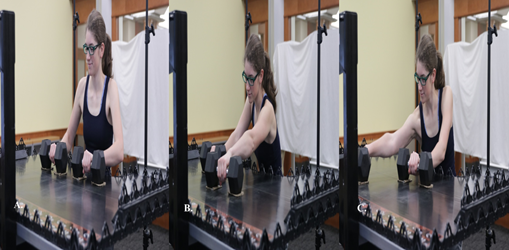
Figure 2: Phases of movement during high-intensity WSKCRT on the FinisherTM.
(A) Neutral position; (B) Concentric phase left arm; (C) Concentric phase right arm
(A) Neutral position; (B) Concentric phase left arm; (C) Concentric phase right arm
Statistical Analyses
Data were analyzed using the Statistical Package for the Social Sciences Statistical version 22.0 (SPSS, IMB Inc., Chicago, IL.). Analyses were performed for all participants who achieved 80% adherence or better. Descriptive statistics were used for demographic information, pre-intervention, and post-intervention measures (means and standard deviation). Percentage of change scores from pre- to post-intervention for all dependent variables were calculated by dividing pre-intervention values into the change scores for the corresponding dependent variable.
Data were analyzed using the Statistical Package for the Social Sciences Statistical version 22.0 (SPSS, IMB Inc., Chicago, IL.). Analyses were performed for all participants who achieved 80% adherence or better. Descriptive statistics were used for demographic information, pre-intervention, and post-intervention measures (means and standard deviation). Percentage of change scores from pre- to post-intervention for all dependent variables were calculated by dividing pre-intervention values into the change scores for the corresponding dependent variable.
Paired samples t-tests were applied to determine the exercise effects of the group for RMR, fat mass, fat-free mass, and BF%. To reduce Type I error risk, post hoc Bonferroni corrections were applied which tested each dependent variable at an alpha level of significance of p ≤ 0.0125. All results are presented as mean ± SD and Cohen’s d effect sizes. An effect size for all statistically significant dependent variables was considered small, medium, and large at the values of 0.2, 0.5, and 0.8, respectively [29].
Demographic | Pre, mean (SD) |
Age (years) | 20.3 (2.6) |
Height (cm) | 174.8 (7.9) |
Body mass (kg) | 80.2 (20.3) |
Table 1: Baseline Characteristics of Participants (n = 6).
Abbreviations: cm, centimeters; kg, kilograms
Abbreviations: cm, centimeters; kg, kilograms
Results and Discussion
All participants completed the training sessions with 100 percent compliance. No physical complications occurred or were reported. Table 1 shows the subjects’ pre-intervention characteristics for age, body mass, and height. Pre- and post-intervention means, SD’s, change, and percentage of change scores for all outcome variables are presented in Table 2.
Variable | Pre, mean (SD) | Post, mean (SD) | Change score, mean | % Change |
RMR (kcal/day) | 1,859.6 (620.6) | 2,271.6 (761.6) | +412 | +22.2% |
Fat-Free Mass (kg) | 61.03 (13.6) | 62.7 (13.7) | +2.67 | +4.4% |
Fat Mass (kg) | 19.2 (9.7) | 16.6 (8.3) | -2.6 | -13.5 |
Body Fat% | 23.1 (8.7) | 20.3 (7.6) | -2.8 | -12.1% |
Table 2: Pre- and Post six Weeks of High-Intensity WSKCRT Outcome Variables (n = 6).
Abbreviations: BF%: Body fat percentage; kcal, kilocalories; kg, kilograms; %, percent.
* Significantly different between pre- and post-intervention (p ≤ 0.0125)
Abbreviations: BF%: Body fat percentage; kcal, kilocalories; kg, kilograms; %, percent.
* Significantly different between pre- and post-intervention (p ≤ 0.0125)
Resting Metabolic Rate
Compared to baseline, RMR increased by an average of 412 kilocalories/day (kcal/day) which was an improvement of 22.2% (Table 2). Following post hoc Bonferroni correction, changes in RMR failed to reach statistical significance (p = 0.336; Figure 3).
Compared to baseline, RMR increased by an average of 412 kilocalories/day (kcal/day) which was an improvement of 22.2% (Table 2). Following post hoc Bonferroni correction, changes in RMR failed to reach statistical significance (p = 0.336; Figure 3).
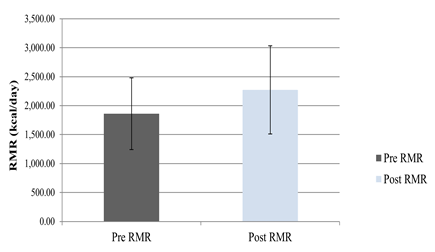
Figure 3: Results of paired samples t-tests, following post-hoc analyses, comparing pre-to-post training RMR mean (±SD) values. *p ≤ 0.0125.
Fat-Free Mass
Compared to baseline, the participants’ fat-free mass increased by an average of 2.67 kg (Table 2). This increase resulted in a 4.4% increase in fat-free mass (Table 2). Following a post hoc Bonferroni correction, the improvement in fat-free mass was not statistically significant (p = 0.028) (Figure 4).
Compared to baseline, the participants’ fat-free mass increased by an average of 2.67 kg (Table 2). This increase resulted in a 4.4% increase in fat-free mass (Table 2). Following a post hoc Bonferroni correction, the improvement in fat-free mass was not statistically significant (p = 0.028) (Figure 4).
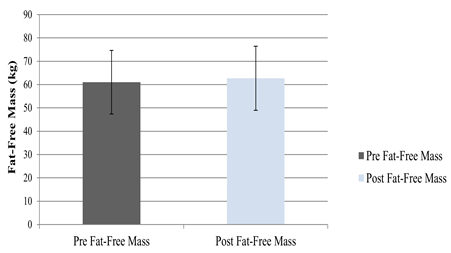
Figure 4: Results of paired samples t-tests, following post-hoc analyses, comparing pre-to-post training fat-free mass mean (±SD) values. *p ≤ 0.0125.
Fat Mass
Fat mass decreased by an average of 2.6 kg (Table 2). This decrease in fat mass was a decline of 13.5% (Table 2). Following a post hoc Bonferroni correction, the decrease in fat mass was statistically significance (p = 0.010; d = 0.288) (Figure 5).
Fat mass decreased by an average of 2.6 kg (Table 2). This decrease in fat mass was a decline of 13.5% (Table 2). Following a post hoc Bonferroni correction, the decrease in fat mass was statistically significance (p = 0.010; d = 0.288) (Figure 5).
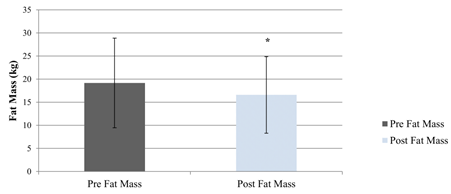
Figure 5: Results of paired samples t-tests, following post-hoc analyses, comparing pre-to-post training fat mass mean (± SD) values. *p ≤ 0.0125.
Body Fat Percentage
Participants’ BF% decreased by an average of 2.8%, which marked a -12.1% percent change from baseline [Table 2]. This decrease resulted in a change score of -12.1% [Table 2]. After post hoc Bonferroni correction, the decrease in BF% was statistically significant (p = 0.004; d = 0.343) (Figure 6).
Participants’ BF% decreased by an average of 2.8%, which marked a -12.1% percent change from baseline [Table 2]. This decrease resulted in a change score of -12.1% [Table 2]. After post hoc Bonferroni correction, the decrease in BF% was statistically significant (p = 0.004; d = 0.343) (Figure 6).
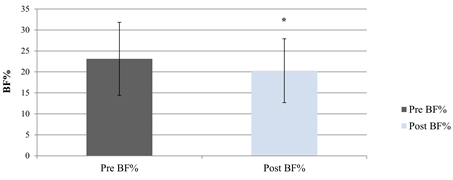
Figure 6: Results of paired samples t-tests, following post-hoc analyses, comparing pre-to-post training body fat percentage (BF %) mean (±SD) values. *p ≤ 0.0125.
Discussion
With epidemiological data indicating a high prevalence of obesity and persons being overweight in the college population combined with the known contributions of poor body composition to negative health outcomes, there is a need to evaluate the efficacy of novel exercise programs and their implications on the main determinants of becoming obese or overweight [1, 2]. In the present pilot study, we evaluated the exercise effects of HILVET using a novel WSKCRT modality, the FinisherTM, on RMR, fat-free mass, fat mass, and BF%. Our results indicate, after six weeks of adherence to our novel HILVET protocol, significant reductions in fat mass (Figure 5) and BF% (Figure 6) were observed among recreationally active college-aged adults. Also, no significant changes were observed for RMR (Figure 3) and fat-free body (Figure 4).
The hallmark of the present pilot study was that six weeks of high-intensity low-volume WSKCRT, using the FinisherTM, was able to significantly reduce fat mass and BF%. Our results are similar to those found by Trapp., et al. [11] who evaluated the impact of a 15-week HILVET cycling program in comparison to a 15-week steady state cycling program on total body and subcutaneous leg and trunk fat among 18-30 year old women. In this HILVET cycling protocol, the participants completed eight seconds of sprinting followed by 12 seconds of light pedaling, repeated up to 60 times per session, for 45 total sessions.
This protocol resulted in significantly greater reductions of total body and subcutaneous fat reduction than steady state cycling. However, it is important to note that the current pilot study did not seek to compare HILVET to a traditional endurance program, but rather to examine the efficacy of a novel form of HILVET on several determinants of obesity and becoming overweight. In this respect, the present pilot study does reveal the potential efficacy of HIVLET utilizing a WSKCRT modality, as our participants saw significant reductions in fat mass and BF% after just six weeks of progressive HILVET.
Our findings are in contrast to those of Mazurek., et al. [30] who determined that BF% was ultimately unchanged following eight weeks of high-intensity cycling among college-aged females. The HILVET protocol used in Mazurek., et al. [30] consisted of participants performing one high-intensity cycling session per week in addition to two 45-minute physical education classes each week for eight weeks. Whereas, in our pilot study, the HILVET protocol was performed three days per week as opposed to one day per week.
Interestingly, Mazurek., et al. [30] found that the supplementation of an additional traditional aerobic endurance protocol per week was more effective at influencing fat loss and body composition than the supplementation of a HILVET protocol. This result is not a novel finding in that others have found traditional aerobic exercise protocols were more effective than HILVET protocols for promoting fat loss and fat free mass [31].
Previous research has suggested several potential mechanisms for induced fat loss following HILVET. One of these proposed mechanisms is an acute increase in plasma catecholamine concentration. Trapp., et al. [32] demonstrated that an acute 20 minute bout of HILVET cycling significantly increased plasma epinephrine and norepinephrine in 19-30 year old trained and untrained females. More recently, Williams., et al. [33] compared the hormonal responses between acute bouts of traditional aerobic exercise and HILVET cycling. Williams., et al. [33] found that plasma epinephrine and norepinephrine concentration was significantly higher following HILVET cycling in comparison to 60 minutes of aerobic exercise performed at 65% VO2 peak. The importance of the increase in the concentration of plasma epinephrine and norepinephrine following HILVET is that the catecholamines are paramount in mediating lipolysis and fatty acid mobilization, a critical process in the reduction of fat mass and BF% [34]. Given the results of the present pilot study, it is plausible that the participants were subject to repeated spikes in plasma epinephrine and norepinephrine, following each high-intensity bout of WSKCRT, thus potentially leading to a significant reduction in BF%. It is also suggested that improvements in maximal mitochondrial oxidative capacity, in response to HILVET, could be a potential mechanism for reductions in body fat. Previous investigations have shown that HILVET significantly increases mitochondrial enzyme activity and content [35]. These adaptations may be beneficial for body fat reduction as liberated fatty acids would be oxidized as opposed to being stored as triglycerides in skeletal muscle or adipose tissue. However, we cannot determine if the reduction in BF% seen in our pilot study correlated to the aforementioned adaptations as they were not directly assessed.
Given that this pilot study revealed statistically significant improvements in fat mass and BF%, we would have expected to see a concurrent improvement in RMR and fat-free mass. This notion is based on previous research findings suggesting that elevations in RMR is a plausible mechanism for inducing positive changes in fat mass and BF% [12] in addition to research indicating a positive association between increases in muscle size and RMR [17, 18]. In the present study, fat-free mass was a measured outcome which consists of, in part, skeletal muscle mass. Improvements in fat-free mass failed to reach statistical significance and thereby it is possible that whole-body skeletal muscle mass did not increase enough to elicit significant improvements in RMR.
Limitations
The predominant limitation to the present pilot study was a small sample size. Additionally, RMR was assessed using the modified Weir equation [26] and did not take into account normalizations in body weight, fat mass, or fat-free mass. Also, on the day of RMR testing, participants walked to the laboratory instead of being transported by the researchers. This may have resulted in RMR estimates being skewed, especially given that half of the participants completed the intervention in the summer and the other half completed the intervention in mid-autumn. Important for future research in this area, consideration must be given to the potential role that previous physical activity plays in RMR adaptations.
The predominant limitation to the present pilot study was a small sample size. Additionally, RMR was assessed using the modified Weir equation [26] and did not take into account normalizations in body weight, fat mass, or fat-free mass. Also, on the day of RMR testing, participants walked to the laboratory instead of being transported by the researchers. This may have resulted in RMR estimates being skewed, especially given that half of the participants completed the intervention in the summer and the other half completed the intervention in mid-autumn. Important for future research in this area, consideration must be given to the potential role that previous physical activity plays in RMR adaptations.
Research indicates that persons who perform resistance training have greater improvements in RMR [36]. In our study, participants were only required to be recreationally active for inclusion and no regard was given for specific exercise training histories. Next, we could not elucidate the contribution or lack of contribution of the final exercise session on RMR. Prior research demonstrates that following a bout of high-intensity exercise, RMR remains elevated 12-22 hours post-exercise [37,38]. In our study, post-intervention measures were collected 72 hours after the final exercise session; therefore any elevations of RMR in response to high-intensity WSKCRT may have diminished prior to post-intervention testing.
Conclusion
Despite the limitations, our pilot study demonstrated the potential efficacy of high-intensity low-volume WSKCRT using the FinisherTM, a novel WSKCRT modality, for reducing fat mass and BF% among recreationally active college-aged students. Improvements in these particular areas are paramount in warding off or managing overweightness and obesity. These improvements were seen in just six weeks with only a weekly time commitment of 18-40.5 minutes. This may help offset one of the main barriers to exercise participation among college students. Practically, high-intensity low-volume WSKCRT, utilizing the FinisherTM, may be a safe, effective, and a time efficient method of exercise for recreationally active college students seeking to prevent the onset of obesity and improve body composition.
References
- Flegal KM., et al. “Prevalence of obesity and trends in the distribution of body mass index among US adults, 1999-2010.” JAMA 307.5 (2012): 491-497.
- American College Health Association. "American college health association-national college health assessment II: Undergraduate students reference group executive summary spring 2014." Hanover, MD: American College Health Association (2015).
- Cheng HL., et al. "The health consequences of obesity in young adulthood." Current Obesity Reports 5.1 (2016): 30-37.
- Must A., et al. "The disease burden associated with overweight and obesity." JAMA 282.16 (1999): 1523-1529.
- Lazo M., et al. "Prevalence of nonalcoholic fatty liver disease in the United States: the Third National Health and Nutrition Examination Survey, 1988–1994." American Journal of Epidemiology 178.1 (2013): 38-45.
- Yumuk V., et al. "An EASO position statement on multidisciplinary obesity management in adults." Obesity Facts7.2 (2014): 96-101.
- Small M., et al. "Changes in eating and physical activity behaviors across seven semesters of college: living on or off campus matters." Health Education and Behavior 40.4 (2013): 435-441.
- Lee MG., et al. "Resting metabolic rate after endurance exercise training." Medicine and Science in Sports andExercise 41.7 (2009): 1444-1451.
- Heydari M., et al. “The effect of high-intensity intermittent exercise on body composition of overweight young males.” Journal of Obesity 2012 (2012): 1-8.
- Gillen JB., et al. "Is high-intensity interval training a time-efficient exercise strategy to improve health and fitness?" Applied Physiology, Nutrition, and Metabolism 39.3 (2013): 409-412.
- Trapp EG., et al. "The effects of high-intensity intermittent exercise training on fat loss and fasting insulin levels of young women." International Journal of Obesity 32.4 (2008): 684-691.
- Rosenkilde M., et al. "Body fat loss and compensatory mechanisms in response to different doses of aerobic exercise—a randomized controlled trial in overweight sedentary males." American Journal of Physiology-Regulatory, Integrative and Comparative Physiology 303.6 (2012): R571-R579.
- Maehlum S., et al. "Magnitude and duration of excess post exercise oxygen consumption in healthy young subjects." Metabolism-Clinical and Experimental 35.5 (1986): 425-429.
- McAninch EA and Antonio C. Bianco. "Thyroid hormone signaling in energy homeostasis and energy metabolism." Annals of the New York Academy of Sciences 1311.1 (2014): 77-87.
- McMurray RG and Anthony C. Hackney. "Interactions of metabolic hormones, adipose tissue and exercise." Sports Medicine 35.5 (2005): 393-412.
- Sedlock DA., et al. "Excess post exercise oxygen consumption after aerobic exercise training." International Journal of Sport Nutrition and Exercise Metabolism 20.4 (2010): 336-349.
- Sparti A., et al. "Relationship between resting metabolic rate and the composition of the fat-free mass." Metabolism46.10 (1997): 1225-1230.
- Sun M., et al. "Increased BMR in overweight and obese patients with type 2 diabetes may result from an increased fat-free mass." Journal of Huazhong University of Science and Technology [Medical Sciences] 36.1 (2016): 59-63.
- Burgomaster KA., et al. "Similar metabolic adaptations during exercise after low volume sprint interval and traditional endurance training in humans." The Journal of Physiology Society 586.1 (2008): 151-160.
- Gibala MJ., et al. "Short‐term sprint interval versus traditional endurance training: similar initial adaptations in human skeletal muscle and exercise performance." The Journal of Physiology 575.3 (2006): 901-911.
- Hottenrott K., et al. "Effects of high intensity training and continuous endurance training on aerobic capacity and body composition in recreationally active runners." Journal of Sports Science and Medicine 11.3 (2012): 483-488.
- McKay BR., et al. "Effect of short-term high-intensity interval training vs. continuous training on O2 uptake kinetics, muscle deoxygenation, and exercise performance." Journal of Applied Physiology 107.1 (2009): 128-138.
- Palmer T., et al. “Sport-specific training targeting the proximal segments and throwing velocity in collegiate throwing athletes.” Journal of Athletic Training 50 (2015): 567-577.
- Hoffman MD and Haylee E Donaghe BS. "Physiological responses to body weight–supported treadmill exercise in healthy adults." Archives of Physical Medicine and Rehabilitation 92.6 (2011): 960-966.
- Warburton DE., et al. "The 2014 physical activity readiness questionnaire for everyone (PAR-Q+) and electronic physical activity readiness medical examination (ePARmed-X+)." Health & Fitness Journal of Canada 7.1 (2014): 80-83.
- Weir JB. "New methods for calculating metabolic rate with special reference to protein metabolism." Nutrition 6 (1990): 213-221.
- Jackson AS and Pollock ML. “Practical assessment of body-composition.” The Physician and Sports Medicine 13.5 (1985): 76-90.
- Siri WE. "Body composition from fluid spaces and density: analysis of methods. 1961." Nutrition 9.5 (1993): 480-491.
- Cohen J. “Statistical power analysis for the behavioral sciences”. 2nd edition. Lawrence Earlbaum Associates, 1988.
- Mazurek K., et al. "High intensity interval and moderate continuous cycle training in a physical education programme improves health-related fitness in young females." Biology of Sport 33.2 (2016): 139-144.
- Keating SE., et al. "Continuous exercise but not high intensity interval training improves fat distribution in overweight adults." Journal of Obesity 2014 (2014): 1-12.
- Trapp EG., et al. "Metabolic response of trained and untrained women during high-intensity intermittent cycle exercise." American Journal of Physiology-Regulatory, Integrative and Comparative Physiology 293.6 (2007): R2370-R2375.
- Williams CB., et al. "Changes in mechanisms proposed to mediate fat loss following an acute bout of high-intensity interval and endurance exercise." Applied Physiology, Nutrition, and Metabolism 38.12 (2013): 1-9.
- Arner P. "Catecholamine-induced lipolysis in obesity." International Journal of Obesity 23.1 (1999): 10-13.
- Talanian JL., et al. "Two weeks of high-intensity aerobic interval training increases the capacity for fat oxidation during exercise in women." Journal of Applied Physiology 102.4 (2007): 1439-1447.
- Willis, LH., et al. "Effects of aerobic and/or resistance training on body mass and fat mass in overweight or obese adults." Journal of Applied Physiology 113.12 (2012): 1831-1837.
- Greer BK., et al. "EPOC comparison between isocaloric bouts of steady-state aerobic, intermittent aerobic, and resistance training." Research Quarterly for Exercise and Sport 86.2 (2015): 190-195.
- Paoli A., et al. "High-Intensity Interval Resistance Training (HIRT) influences resting energy expenditure and respiratory ratio in non-dieting individuals." Journal of Translational Medicine 10.1 (2012): 237.
Citation:
Matthew D McCabe., et al. “Changes in Resting Metabolism, Body Fat, and Fat-Free Mass among College Students in Response
to Novel High-Intensity Weight-Supported Exercise”. Orthopaedic Surgery and Traumatology 2.4 (2018): 356-366.
Copyright: © 2018 Matthew D McCabe., et al. This is an open-access article distributed under the terms of the Creative Commons Attribution License, which permits unrestricted use, distribution, and reproduction in any medium, provided the original author and source are credited.