Research Article
Volume 3 Issue 6 - 2019
Analysing Push-out Bond Strength of ProRoot MTA, Biodentine & GIC used During Repair of Furcal Perforations.
Department of Conservative Dentistry and Endodontics, Penang International Dental College, Butterworth, Malaysia
*Corresponding Author: Akarsha Singh Multani, Bachelor of Dental Surgery, Master of Dental Surgery (Conservative Dentistry and Endodontics) Lecturer, Department of Conservative Dentistry and Endodontics, Penang International Dental College, Level 18, NB Tower, Jalan Bagan Luar 5050, Butterworth 12000, Penang, Malaysia.
Received: May 08, 2019; Published: June 10, 2019
Abstract
Background: Furcal perforation is usually an undesired complication that can occur due to caries, resorption or iatrogenically, during opening of endodontic access cavities or exploring canal orifice of multirooted tooth. They can lead to the failure of endodontic treatment and thus, their repair is deemed necessary. Therefore, the objective of this research was to compare and evaluate the push-out bond strength and its time-dependent change, of various materials when used for furcal perforations i.e. change in strength after 24 hours and 7 days to determine which material had the maximum push-out bond strength measurement so as to bear the forces of compaction of the coronal restoration.
Materials and Methods: The study was carried out by preparing standard endodontic access cavities and decoronating 60 molars 5mm above the pulpal floor. After which, perforations were made in the furcal area of the pulpal floor. The size of the perforations was measured with a Stereomicroscope and ImageJ analysis. The remaining dentin thickness was measured with digital calipers. In each sample, a saline-soaked sponge was placed apical to the furcal perforation. For stabilisation, the roots were embedded in Silicon putty impression material to simulate the periodontal ligament, the apical third being embedded in cold-cure acrylic. The perforations were repaired with 3 different materials, Group I Pro Root White MTA, Group II Biodentine and Group III GIC. Out of 20 samples, 10 samples in each group were subjected to push-out bond strength measurement after 24 hours & the remaining 10 after 7 days. Universal Instron testing machine with 1-mm-thick cylindrical stylus was used for push-out bond strength measurement at a crosshead speed of 0.5 mm/min. Statistical analysis was done using One-way Anova, Post Hoc multiple comparison test and t Test.
Results: Decreasing order of strength: Biodentine > MTA> GIC
Conclusion: Biodentine is the most appropriate material for functionally restoring a tooth in lesser time and providing more strength against the forces of coronally restoring the tooth.
Key words: Furcal perforation push-out bond strength
Introduction
Furcal perforation is usually an undesired complication that can occur iatrogenically during opening of endodontic access cavities or exploring canal orifice of multirooted tooth. They may also manifest as a result of caries or resorption. [1] The American Association of Endodontists (AAEs) Glossary of Endodontic Terms defines perforation as the mechanical or pathological communication between the root canal system and the external tooth surface. [2]
Perforations are the second greatest cause of endodontic failure and Ingle JI has accounted for 9.6% of all unsuccessful cases to perforations. [3] Perforations are treatable, that is why, on the basis of the factors impacting the outcome of treatment, Fuss Z & Trope M have classified root perforations as fresh, old, small, large, coronal, crestal and apical perforations. [1] Larger, supracrestal, strip-type perforations with bone loss and delayed repair have the most guarded prognosis. Conversely, small, subcrestal, furcal-type perforations with little bone loss and immediate repair have the best prognosis. [1,3] It’s advisable to repair the perforation as soon as it is identified since any delay will allow the bacterial ingress leading to an endodontic-periodontal lesion.[4]
Perforations occur most frequently on the floor of the pulp chamber because of presence of accessory canals and less remaining dentin thickness. Often, they result in secondary periodontal involvement with eventual loss of teeth. Therefore if a technique for treating these perforations could be perfected, many teeth could be saved. [5]
Furcal perforations are comparatively less difficult to treat than root perforations because of ease of access and visibility to the operator. [3,6] Although, Cohen S and Burns RC had stated that the major difficulty with nonsurgical repair of a mechanical perforation is that the defect acts as a bottomless pit. Control of the repair material is difficult, and extrusion of the filling material into the periodontal ligament space is common. [5]
The main goal of furcal perforation repair is to quickly seal the perforation site and provide optimal conditions for periodontal reattachment at the area. This goal can only be achieved if a complete seal is obtained. Selection of the restorative material for this purpose is an important factor in determining the prognosis of the perforated tooth. [7]
An ideal perforation repair material should provide an adequate seal, be biocompatible, dimensionally stable, insoluble, not affected by blood contamination, bactericidal, induce osteogenesis and healing, induce mineralization and cementogenesis, be radiopaque, allow easy manipulation and facilitate non-surgical placement. [4,8] Along with these, the material should have an adequate pushout strength so as to bear the condensation force to which it will be subjected during placement of an intracoronal restoration. [4]
Since the perforation site will be in direct communication with the periodontal area, it will be difficult to achieve a completely dry field. Delivanis PD., et al. (1981) had quoted that sites in the coronal third of the root or through the floor of the pulp chamber are in closer proximity to the gingival sulcus where if the perforation is not sealed immediately, it would result in a continuous irritation to the area leading to a communication to the gingival sulcus and eventual uncorrectable periodontal defect. Therefore, the material should have hydrophilic properties. [6]
Glass ionomer cements (GIC) were first used in 1990 to repair lateral root perforations [3] with the advantage of GIC chemically bonding to the tooth, releasing fluoride and calcium/strontium ions providing this material with the potential for remineralization of carious tissues, where ion exchange could replenish the demineralized tissues’ ions, thus tipping the balance in favor of apatite formation. [9]
However, GIC and other restorative materials such as amalgam, composites, and zinc oxide eugenol cements require the challenge of securing a dry field in a bleeding perforation site. In addition, none of these can promote new tissue formation. Newer materials like Mineral Trioxide Aggregate (grey and white), demineralized freeze-dried bone, Bioaggregate, Biodentine, are bio-compatible and thought to promote osteogenesis. [10]
Mineral trioxide aggregate (MTA) was developed at Loma Linda University, in the 1993, as a root-end filling material. [11] MTA, introduced by Torabinejad M, is capable of creating a thorough seal between the root canals and external dental surfaces. In vitro and in vivo studies have shown that MTA has considerable sealing ability and marginal adaptation. The main components of MTA are calcium and phosphate ions. Since these elements are also the main components of dental hard tissues, this may contribute to the biological compatibility of MTA with surrounding tissues. [12] One of the main disadvantages of MTA is the long setting time of 2 hours and 45 minutes. Also it has a low compressive strength at 24 hrs of mixing, that is, 40 MPa, which increases to 67 MPa after 21 days. [13]
To overcome these disadvantages, Biodentine has been introduced. It is composed of a mixture of 5µm round particles embedded in a calcium silicate hydrate matrix. Smaller zirconium oxide particles are also present which have high electron contrast. It has a reduced setting time of around 12 min considering MTA. The compressive strength of Biodentine is around 100 MPa in the first hour, which continues to improve until it reaches to 300 MPa after 1 month. [14]
Undeniably, the tooth undergoing treatment will be restored coronally after the furcal perforation has been appropriately rectified. Hence, the present study aims to compare the push-out bond strength of two calcium silicate based materials, that is, MTA and Biodentine, used for repair of furcal perforations in molars alongwith conventional GIC, utilizing Universal Instron Testing Machine. The before mentioned strength parallels the condensation force required for a coronal restoration. Also, the study evaluated the difference in the strength after setting times of 24 hours and 1 week.
Materials and Methods
Freshly extracted 60 intact human permanent maxillary and mandibular molars were collected from the Department of Oral and Maxillofacial Surgery of Swami Devi Dyal Hospital and Dental College, Panchkula and adjoining clinics. While collecting the samples no consideration was given to the age and sex of the patients. All the selected teeth were cleaned mechanically to remove periodontal tissue and bone, washed and stored in distilled water. Teeth with cracks, open apices, root caries, or fused roots were excluded from the study.
A standardised endodontic access cavity was prepared in all the samples using an EndoAccess bur. Using a William’s periodontal probe, 5 mm from the pulpal floor was measured and marked with a felt tip pen on the external coronal surface of the sample. The teeth were decoronated at the level marked previously, (Figure 1) with a diamond sectioning disk, which was water cooled using a three-way syringe.
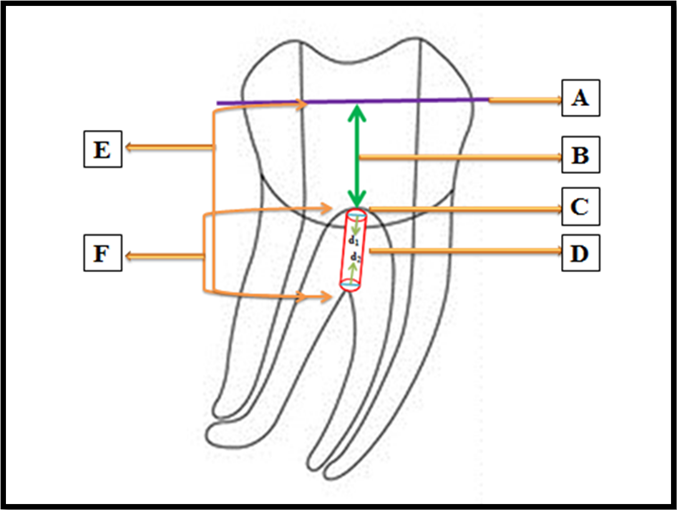
Figure 1: A- Decoronation level of a sample in which access cavity had been prepared.
B- 5 mm measured with William’s periodontal probe from the pulp chamber floor to the level at which the sample was decoronated.
C- Pulp chamber floor.
D- Furcal perforation with the coronal diameter in the pulpal floor represented by d1 and apical diameter in the furcation area of the roots
E- The length measured by the digital callipers from the decoronated surface of the crown of the sample to the furcal area represented by H.
F- The remaining dentin thickness from the pulp chamber floor to the furcal area calculated by decreasing 5 mm from the reading obtained by the digital callipers as represented by h.
B- 5 mm measured with William’s periodontal probe from the pulp chamber floor to the level at which the sample was decoronated.
C- Pulp chamber floor.
D- Furcal perforation with the coronal diameter in the pulpal floor represented by d1 and apical diameter in the furcation area of the roots
E- The length measured by the digital callipers from the decoronated surface of the crown of the sample to the furcal area represented by H.
F- The remaining dentin thickness from the pulp chamber floor to the furcal area calculated by decreasing 5 mm from the reading obtained by the digital callipers as represented by h.
In each sample, a furcal perforation was prepared with a high-speed long shank round bur, E0123 010 with an attempt to centralize the perforation in the apical aspect of the furcal area between the roots. Since there could be a discrepancy in the coronal and apical diameters of the furcal perforations within the same sample and as compared with other samples, each perforation was photographed coronally and apically by a Stereomicroscope (Figure 2).
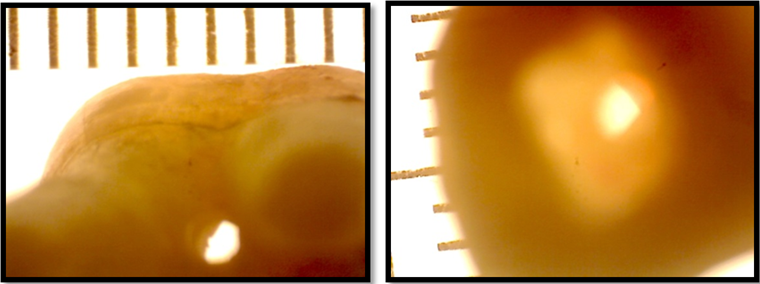
Figure 2: Stereomicroscopic images of furcal perforation as viewed left: apically, right: coronally.
The images obtained were analyzed by ImageJ software for the purpose of measuring the coronal and apical diameter (d1 and d2 respectively) of each perforation in all the samples. From the diameter, the coronal and apical radius (r1 and r2 respectively) was deduced by dividing the diameter by 2. Inclusively, the length from the decoronated surface of the crown to the furcal area of the roots was measured with micrometer digital callipers represented by (H). (Figure 1)
The remaining dentin thickness (h) from the pulpal floor to the furcal area of the roots was calculated by reducing 5 mm from the length measured by the micrometer digital callipers as the crown of each tooth had been decoronated at a length of 5 mm from the pulpal floor. Before repairing each sample, as per the measurements of the stereomicroscope, the furcal perforation had been considered as truncated cone. (Figure 3)
The push-out bond strength of the repair material depends directly upon the force applied and inversely upon the lateral surface area of the perforation.
The Lateral surface area or Adhesion area of each perforation was calculated as follows:
π L(r1 + r2)
where
π is the constant 3.14,
r1 is the coronal larger radius,
r2 is the apical smaller radius
and L is calculated as follows:
L= √(r1 – r2)2 + h2
where h is the thickness of the repair material
π L(r1 + r2)
where
π is the constant 3.14,
r1 is the coronal larger radius,
r2 is the apical smaller radius
and L is calculated as follows:
L= √(r1 – r2)2 + h2
where h is the thickness of the repair material
Following the simulation of the periodontium, the samples were randomly divided into three major experimental groups of twenty samples each, based on the repair material used, which were:
- GROUP I: Furcal perforations repaired using Pro-Root White MTA
- GROUP II: Furcal perforations repaired using Biodentine
- GROUP III: Furcal perforations repaired using Glass Ionomer Cement.
All the samples were stored in 100% humidity before being mounted in loading fixtures using rapid repair acrylic resin and iron molds. One millimetre thick cylindrical stylus was placed coronally on the repaired perforation in each sample and a force was applied by the Universal Instron Testing Machine (UTM), at a crosshead speed of 0.5 mm/min in a coronal-apical direction. The tip of the stylus was positioned so that it only contacted the repair material
Push-out bond strength (In MegaPascals) = Force at which the perforation repair material failed (In Newtons)
Lateral surface area of the furcal perforation (in mm2)
Lateral surface area of the furcal perforation (in mm2)
The bonded (adhesion) surface area of each section was calculated as follows: [15, 16]
π L(r1 + r2)
where
r2 is the apical smaller radius
and L is calculated as follows: [16]
L= √(r1 – r2)2 + h2
where h is the thickness of the repair material
π L(r1 + r2)
where
r2 is the apical smaller radius
and L is calculated as follows: [16]
L= √(r1 – r2)2 + h2
where h is the thickness of the repair material
Lastly, the results obtained in the present study were statistically analyzed by One-way ANOVA, Post Hoc multiple comparison test and t-test.
Results
- The maximum push-out bond strength was observed at 7 days for Biodentine.
- At 24 hours, there was statistical difference among the three materials, with the highest to lowest push-out bond strength as follows: Biodentine > ProRoot White MTA > Glass ionomer cement.
- At 7 days, there was statistical difference among the three materials, with the highest to lowest push-out bond strength as follows: Biodentine > ProRoot White MTA > Glass ionomer cement.
- On comparing subgroups of Group I ProRoot White MTA, the push out bond strength of subgroup IA (setting time 24 hours) was significantly less than IB (setting time 7 days).
- Furthermore, upon comparing subgroups of Group II Biodentine, the push-out bond strength of subgroup IIA (setting time 24 hours) was significantly less than IIB (setting time 7 days).
- In contrast, the comparison of the subgroups of Glass ionomer cement revealed that the push-out bond strength of subgroup IIIA (setting time 24 hours) was significantly more than IIIB (setting time 7 days).
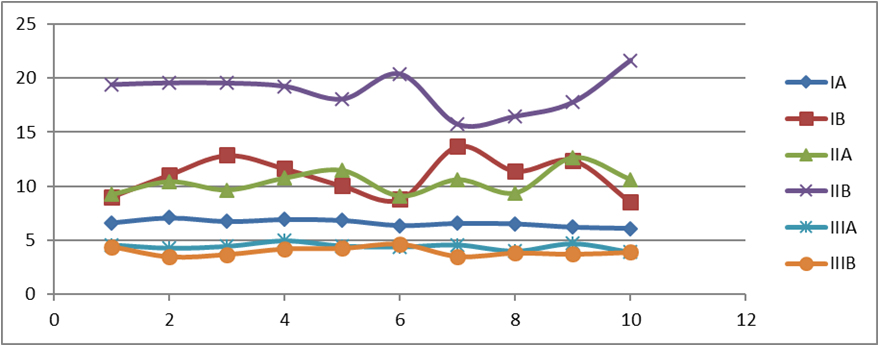
Graph 1: The above scatter diagram displays the push-out bond strength of each sample of every subgroup tested in the present study pertaining to Table no. 1. The highest values have been recorded for Subgroup IIB Biodentine after 7 days.
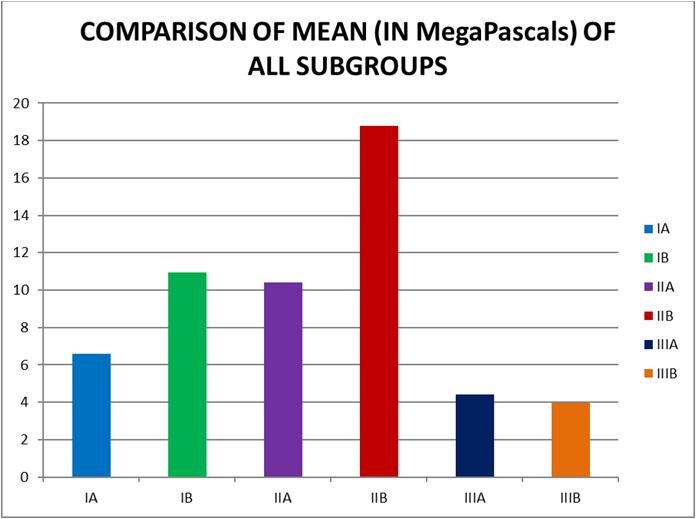
Graph 2: Table no. 2 presents the mean and standard deviation values of all the groups. The push-out bond strength from highest to the lowest at 24 hours and 7 days is as follows: Biodentine > ProRoot White MTA > Glass ionomer cement.
S. No. | Pro Root White MTA | Bio dentine | Glass Ionomer Cement | |||
IA | IB | IIA | IIB | IIIA | IIIB | |
1 | 6.62 | 9.02 | 9.23 | 19.4 | 4.58 | 4.4 |
2 | 7.09 | 11.07 | 10.42 | 19.56 | 4.28 | 3.47 |
3 | 6.74 | 12.86 | 9.65 | 19.54 | 4.44 | 3.68 |
4 | 6.94 | 11.62 | 10.77 | 19.24 | 4.94 | 4.19 |
5 | 6.85 | 10.02 | 11.47 | 18.07 | 4.47 | 4.24 |
6 | 6.36 | 8.77 | 9.08 | 20.38 | 4.38 | 4.63 |
7 | 6.57 | 13.7 | 10.61 | 15.75 | 4.57 | 3.5 |
8 | 6.52 | 11.41 | 9.38 | 16.49 | 4.01 | 3.81 |
9 | 6.2 | 12.4 | 12.68 | 17.78 | 4.68 | 3.7 |
10 | 6.09 | 8.51 | 10.63 | 21.64 | 3.9 | 3.88 |
MEAN | 6.5980 | 10.9380 | 10.3920 | 18.7850 | 4.4250 | 3.9500 |
S.D. | .32027 | 1.80377 | 1.12008 | 1.77797 | .30704 | .39432 |
RANGE | 6.09-7.09 | 8.51-13.70 | 9.08-12.68 | 15.75-21.64 | 3.90-4.94 | 3.47-4.63 |
Table 1: Push-out bond strength of Proroot white MTA, Biodentine and Glass ionomer cement when used for repairing furcal perforations after 24 hours and 7 days in megapascals.
SUBGROUP NO. | NO. OF SAMPLES | MEAN | S.D. ± |
IA | 10 | 6.598 | 0.32027 |
IB | 10 | 10.938 | 1.80377 |
IIA | 10 | 10.392 | 1.12008 |
IIB | 10 | 18.785 | 1.77797 |
IIIA | 10 | 4.425 | 0.30704 |
IIIB | 10 | 3.95 | 0.39432 |
Table 2: Comparison of the mean of all subgroups.
Discussion
The results of the present study showed that the push-out bond strength from the highest to the lowest in the order of: Group IIB (Biodentine after 7 days, 18.785 ± 1.77797 MPa) > Group IB (ProRoot White MTA after 7 days, 10.938 ± 1.80377 MPa) > Group IIA Biodentine after 24 hours, 10.392 ± 1.12008 MPa) > Group IA (ProRoot White MTA after 24 hours, 6.598 ± 0.32027 MPa) > Group IIIA (Conventional Glass ionomer cement after 24 hours, 4.425 ± 0.30704 MPa) > Group IIIB (Conventional Glass Ionomer cement after 7 days, 3.95 ± 0.39432 MPa). After the data was analysed using One-way Anova, Post Hoc multiple comparison test and t-test, the difference between all the subgroups was found to be statistically significant.
The current study showed that the samples restored with ProRoot White MTA had a push-out bond strength of 6.598 ± 0.32027 MPa after a setting time of 24 hours. The strength significantly increased to 10.392 ± 1.12008 MPa after the samples were allowed to set for 7 days. Hashem AA and Wanees Amin SA (2012) reported a push-out bond strength of 8.49 ± 1.75 MPa when MTA was allowed to set for 4 days in contact with phosphate-buffered saline. [17] Saghiri MA., et al. (2010) demonstrated that pH has an effect on the push-out bond strength of MTA in the lumen of root slices, and reported a mean push-out bond strength of 9.46 ± 0.63 MPa when the samples were stored at a pH of 8.4 for 3 days. [18]
In the present study, the dislodgement resistance of MTA was less than Biodentine and more than Conventional Glass ionomer cement at 24 hours setting time. According to Aggarwal V., et al. (2013), this could be attributed to a prolonged maturation process because of the formation of passivating trisulfate layer over hydrating crystals of MTA. [4]
MTA has a long setting time of 165 ± 5 min. Addition of CaCl2 has shown to decrease its setting time. CaCl2 has a negative effect on the compressive strength of MTA. Biodentine is a CSM-based material that has polycarboxylate-based hydrosoluble polymer system described as “water reducing agent” to reduce the overall water content of the mix, along with CaCl2 as a setting accelerator. The combined effect reduces the setting time to 12 min and increases the compressive strength. Because of its high compressive strength, manufacturers recommend Biodentine to be used as an interim restoration or as dentine substitute under composite restorations, apart from other endodontic uses. [4] In the present study, Biodentine had a significantly higher push-out bond strength, i.e. 10.938 ± 1.80377 MPa, than MTA and Conventional GIC (4.425 ± 0.30704 MPa) after 24 hours setting time.
After 7 days, MTA had a statistically significant lower push-out bond strength (10.938 ± 1.80377 MPa) than Biodentine (18.785 ± 1.77797 MPa) and significantly higher than Glass ionomer cement (3.95 ± 0.39432 MPa). Exceptionally, the samples restored with Glass ionomer cement had a shown dislodgement resistance less than the average force recorded by Lussi A et al during amalgam condensation (3.7 ± 1.3 MPa). This could be associated with the reason that all samples being contaminated with saline during the formation of the cross-linked matrix could have caused dissolution of the matrix forming cations and anions to the surrounding areas. [19] Even more so, Yao K., et al. (1990) have also reported a detrimental effect on GICs due to moisture contamination. [20] Therefore, Conventional GIC has to be protected against water changes during placement and a few weeks after, if possible. [19,20]
The results of the present study are in concurrence with Aggarwal V., et al. (2013) who found significantly higher push-out bond strength for Biodentine as compared to ProRoot MTA after a setting period of 24 hours. [4]
Moreover, the results are also in concordance with Akcay H., et al. (2016) who had evaluated the push-out bond strength of root-end placed Mineral trioxide aggregate and Biodentine in the absence/presence of blood contamination after a setting period of 1 week. They observed that in the absence of blood contamination, Biodentine (4.01 ± 0.88 MPa) had a significantly higher bond strength than MTA (1.81 ± 1.24 MPa). In the presence of blood, the strength decreased with Biodentine having significantly higher strength (2.07 ± 0.71 MPa) than MTA (0.60 ± 0.45 MPa). [21]
Conjointly, the results are in accordance with Nagas E., et al. (2016) who had evaluated the effect of medicaments on the push-out bond strength of ProRoot MTA and Biodentine resulting in Biodentine (2.59±0.38 MPa, no intracanal medicament) having a significantly higher push-out strength than MTA (2.09±0.34 MPa) after a period of 1 week. [22]
Additionally, the results are similar to those of Guneser MB., et al. (2013) who studied the effect of Various Endodontic Irrigants on the Push-out Bond Strength of Biodentine and Conventional Root Perforation Repair Materials and observed a significantly higher strength for Biodentine (7.12 ± 3.10 MPa) than MTA (3.03 ± 1.28 MPa) after a period of 48 hours. [23] Biodentine was more resistant to dislodgement forces than MTA in the present study after 7 days. In their study, saline-treated MTA samples resisted dislodgement that indicated that the compressive strength of MTA increased when immersed in saline solutions because of the remaining unreacted mineral oxides, which may be solidified after additional supplied hydration and may result in the increased strength of the material. The biomineralization ability of Biodentine, having a smaller particle size, most likely through the formation of tags, may be the reason of the dislodgement resistance. [23] Han L and Okiji T (2013) showed that calcium and silicon ion uptake into dentin leading to the formation of tag-like structures in Biodentine was higher than MTA. [24]
In contrast, Aggarwal V., et al. (2013) observed a statistically non-significant difference between the push-out bond strength of Biodentine and ProRoot MTA when used to repair furcal perforations, after a period of 1 week. [4]
Furthermore, Makkar S., et al. (2015) evaluated push-out bond strength in dentin slices of extracted single–rooted human teeth and reported the highest push-out bond strength for GIC in neutral medium, that is, GIC (33.22 MPa) than Biodentine (21.76 MPa) and MTA (8.92 MPa). Also, in acidic medium, GIC (26.75 MPa) had higher push-out bond strength than Biodentine (12.64 MPa) and MTA (2.78 MPa). In alkaline medium, Biodentine performed the best at a strength of 28.89 MPa in comparison to GIC (13.78 MPa) and MTA (8.03 MPa). [25]
Similarly, Yahya MM (2015) evaluated the effect of few endodontic irrigants on the push-out bond strength Biodentine in comparison with Mineral trioxide aggregate (MTA) and Glass ionomer cement (GIC) and found that the push-out bond strength before exposure to irrigating solution in the highest to lowest order, was GIC (9.77 ± 1.19 MPa)> Biodentine (7.77 ± 0.66 MPa)> MTA (3.74 ± 0.57 MPa). After irrigating with saline, the push-out bond strength from highest to lowest was in the following order: GIC (10.02 ± 1.83)> Biodentine (7.70 ± 0.61 MPa)> MTA (3.40 ± 1.66 MPa). The before-mentioned results were statistically significant. [26]
Even more so, Üstün Y., et al. (2015) have found that Biodentine (4.36 ± 2.55 MPa) had less dislocation resistance than ProRoot MTA (8.3 ± 3.9 MPa) in blood contaminated furcal perforations after a period of 14 days. [27]
An important point to be noted is that the materials were tested after their setting in the present study, which is not the real clinical scenario where the tooth is immediately subjected to masticatory stresses. It can be concluded from the results of the present study that the material used for perforation repair should not be negatively affected by moisture contamination. Group III (Glass ionomer cement) showed the minimum push-out bond strength which decreased after 7 days, which grades Group II (Biodentine) and Group I (ProRoot White MTA) as superior materials for repair of furcal perforations. But for clinical relevance, further in-vivo studies with a larger sample size are required.
Conclusion
Within the limitations of the present study, it can be concluded that:
- Glass ionomer cement may not be a suitable material for a furcal perforation due to the moisture contamination provided by the bottomless cavity.
- It would be more appropriate to rectify furcal perforations with calcium silicate materials, i.e. MTA and Biodentine which are affected by continuous exposure to fluids.
- The strength of MTA and Biodentine increased after 7 days, so they are less likely to dislodge while restoring the tooth coronally.
- The push-out bond strength of Biodentine was significantly higher than Pro-Root White MTA both at 24 hours and 7 days. On that account, Biodentine is the most appropriate material for functionally restoring a tooth in lesser time and providing more strength against the forces of coronally restoring the tooth.
A definite conclusion as to which material should be preferred between ProRoot White MTA, Biodentine and Glass Ionomer cement, can be withdrawn after correlating the findings of the present study with clinical research.
Conflict Of Interest
No potential conflict of interest relevant to this article was reported.
No potential conflict of interest relevant to this article was reported.
References
- Tsesis I and Fuss Z. “Diagnosis and treatment of accidental root perforations”. ENDODONTIC TOPICS 13 (2006): 95–107.
- American Association of Endodontists. Glossary of endodontic terms. 7th ed. Chicago: American Association of Endodontists; 2003.
- Gluskin AH, Peters CI, Ruddle CJ, Ralan MW. Retreatment of non-healing endodontic therapy and management of mishaps. In: Ingle JI, Bakland LK, Baumgartner JC, editors. Ingle’s Endodontics. 6th ed. Delhi: CBS Publishers & Distributors; (2013): 1054-88.
- Aggarwal V., et al. “Comparative evaluation of push-out bond strength of ProRoot MTA, Biodentine and MTA Plus in furcation perforation repair”. J Conserv Dent 16.5 (2013): 462–5.
- Alhadainy HA and Himel VT. “Evaluation of the sealing ability of amalgam, Cavit, and glass ionomer cement in the repair of furcation perforations”. Oral Surg Oral Med Oral Pathol 75.3 (1993): 362-6.
- Delivanis PD and Goerig AC. “Repair of Perforations”. Quintessence Int Dent 12.9 (1981): 985-92.
- Saberi E., et al. “Comparison of the Microleakage of Octacalcium Phosphate and Mineral Trioxide Aggregate for Furcal Perforation Repair: An In-Vitro Study”. J Dent Sch Shahid Beheshti Univ Med Sci 31.3 (2013): 129-35.
- Sinkar RC., et al. “Comparison of sealing ability of ProRoot MTA, RetroMTA, and Biodentine as furcation repair materials: An ultraviolet spectrophotometric analysis”. J Conserv Dent 18.6 (2015): 445-8.
- Watson TF., et al. “Present and future of glass-ionomers and calcium-silicate cements as bioactive materials in dentistry: biophotonics-based interfacial analyses in health and disease”. Dent Mater 30.1 (2014): 50-61.
- VanderWeele RA., et al. “Effect of blood contamination on retention charactersistics of MTA when mixed with different liquids”. J Endod 32.5 (2006): 421-4.
- Camilleri J. “The chemical composition of mineral trioxide aggregate”. J Conserv Dent 11.4 (2008): 141–3.
- Shahi S., et al. “Sealing ability of mineral trioxide aggregate and Portland cement for furcal perforation repair: a protein leakage study”. J Oral Sci 51.4 (2009): 601-6.
- Torabinejad M., et al. “Physical and Chemical Properties of a New Root-End Filling Material”. J Endod 21.7 (1995): 349-53.
- Arora V., et al. “Bioactive dentin replacement”. IOSR-JDMS 12.4 (2013): 51-7.
- Patierno JM., et al. “Push-out strength and SEM evaluation of resin composite bonded to internal cervical dentin”. Endod Dent Traumatol 12.5 (1996): 227-36.
- Muvdi BB., et al. Statics for Engineers. 1st ed. New York: Springer-Verlag; Chapter 10, Centers of Gravity, Centers of Mass and Centroids; (1997): 671-9.
- Hashem AA and Wanees Amin SA. “The effect of acidity on dislodgement resistance of mineral trioxide aggregate and bioaggregate in furcation perforations: an in vitro comparative study”. J Endod 38.2 (2012): 245-9.
- Saghiri MA., et al. “Push-out bond strength of mineral trioxide aggregate in the presence of alkaline pH”. J Endod 36.11 (2010): 1856-9.
- Shen C. Dental Cements. In: Anusavice KJ, editor. “Phillips’ Science of Dental Materials. 11th ed”. New Delhi: Elsevier (2007): 471-84.
- Yao K., et al. “Effect of water isolation and early finishing on hardness of glass ionomer cements”. J Osaka Dent Univ 24.2 (1990): 141-7.
- Akcay H., et al. “Evaluation of the bond strength of root-end placed mineral trioxide aggregate and Biodentine in the absence/presence of blood contamination”. Eur J Dent 10.3 (2016): 370-5.
- Nagas E., et al. “Effect of several intracanal medicaments on the push-out bond strength of ProRoot MTA and Biodentine”. Int Endod J 49.2 (2016): 184-8.
- Guneser MB., et al. “Effect of various endodontic irrigants on the push-out bond strength of biodentine and conventional root perforation repair materials”. J Endod 39.3 (2013): 380-4.
- Han L and Okiji T. “Bioactivity evaluation of three calcium silicate based endodontic materials”. Int Endod J 46.9 (2013): 808-14.
- Makkar S., et al. “The effect of altered pH on push-out bond strength of biodentin, glass ionomer cement, mineral trioxide aggregate and Theracal”. Stomatol Glas Srb 62.1 (2015): 7-13.
- Yahya MM. “The Effect of Root Canal Irrigants on The Push–out Bond Strength of Biodentine”. Tikrit J Dent Sci 1 (2015): 69-75.
- Üstün Y., et al. “The effect of blood contamination on dislocation resistance of different endodontic reparative materials”. J Oral Sci 57.3 (2015): 185-90.
Citation:
Akarsha Singh Multani. “Analysing Push-out Bond Strength of ProRoot MTA, Biodentine & GIC used During Repair of Furcal Perforations.” Oral Health and Dentistry 3.6 (2019): 760-770.
Copyright: © 2019 Akarsha Singh Multani. This is an open-access article distributed under the terms of the Creative Commons Attribution License, which permits unrestricted use, distribution, and reproduction in any medium, provided the original author and source are credited.